Re-growing adult organs: mothers do it best
Posted by Tomotsune Ameku, on 30 April 2025
Reproduction is a metabolically costly process. Therefore, the proper regulation of physiological changes in the mother during pregnancy and lactation is crucial for fetal development and neonatal growth. In many mammals, including humans, pregnancy induces systemic changes in hormonal, metabolic, and immune functions.1 Reproductive-associated changes are also known to occur at the organ level, in particular, an increase in intestinal size during pregnancy and lactation has been observed in several mammals such as mice, rats, sheep, and pigs.2,3 However, despite the first report of this phenomenon, documented in 1939,4 the molecular mechanisms underlying maternal intestinal remodelling during reproduction, as well as their physiological significance, have only recently begun to be investigated.5
Studies using fruit flies have shed light on the molecular mechanisms regulating reproductive plasticity and sex differences in adult digestive tract. 6–13 Instead of studying the fly, we decided to focus on the maternal intestinal growth in mice.
Characterising maternal gut growth in epithelium
We first examined the organ size of the small intestine during reproduction and observed that the maternal small intestine is significantly longer and heavier than that of non-pregnant female mice, consistent with previous studies.14,15 Notably, the small intestine remains elongated even one month after weaning, suggesting that gut elongation is an irreversible (or only partially reversible) process.
Next, we investigated the mucosal morphology of the small intestine, which consists of absorptive villi (finger-like projections into the lumen) and crypts (which house progenitor cells). We observed that both villi and crypts increased in size during pregnancy and lactation (Figure 1). Since the size of the crypt-villus structure can be determined by the number and size of epithelial cells—or both—we focused on epithelial proliferation. The intestinal epithelium has one of the fastest turnover rates among all tissues, typically renewing every 3–5 days.
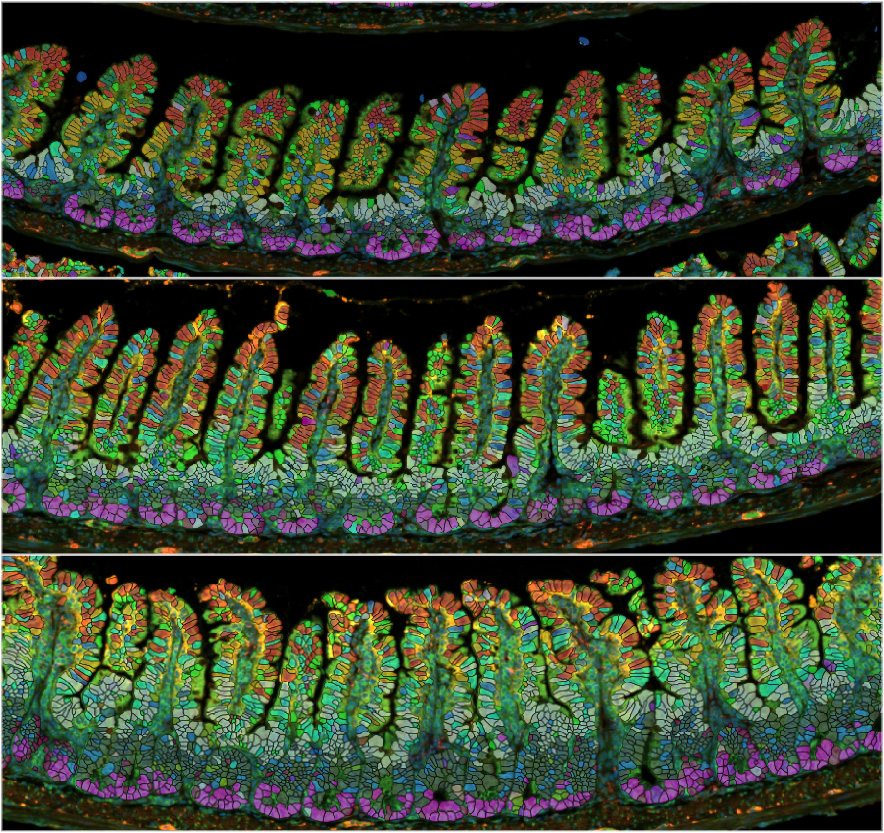
We found that this turnover rate was accelerated during reproduction, with lactating mice exhibiting the highest turnover rate—renewing in just 1.3 days. In contrast to the irreversible elongation of the gut tube, the growth of the gut epithelium is reversible: both the crypt-villus structure and epithelial turnover returned to pre-pregnancy levels within one week after weaning. These results suggest that gut tube elongation and epithelial growth are regulated by different mechanisms.
Remodelling of epithelial cell types in the gut
We have two hypotheses of how the crypt-villus structure enlarges:
- Do epithelial cells increase proportionally across different cell types at a similar rate?
- Or do epithelial cells increase preferentially in specific cell types?
To address this question, we first analysed our single-cell RNA sequencing data from FACS-sorted intestinal epithelial cells, where we did not observe significant differences in cell composition between reproductive and non-reproductive mice. However, since it was unclear whether this lack of difference was due to technical—such as the dissociation of epithelial cells and the FACS-sorting process—or biological reasons, we decided to perform a spatial imaging analysis using the Xenium in situ platform. We profiled subtypes of intestinal epithelial cells—including absorptive enterocytes, secretory cells, and progenitor cells—to compare cell composition between intestines from reproductive and non-reproductive mice.
We actually found that lactating mice had significantly more progenitor cells, specifically isthmus progenitors in the upper crypts, along with their neighbouring enterocytes located at the base of the villi. This indicates that epithelial cell expansion during reproduction occurs preferentially in specific cell types.
While the reason for this preferential increase is still unclear, we speculate that the expansion of the progenitor population supports the accelerated epithelial turnover observed during lactation.
What is the molecular mechanism behind this?
To uncover potential drivers of maternal gut growth, we focused on a transporter gene called SGLT3a, whose expression was upregulated in our transcriptomics datasets—including whole small intestine tissue, FACS-sorted intestinal epithelial cells, and spatial imaging analysis—during reproduction. SGLT stands for sodium-glucose cotransporter, but previous studies have shown that, unlike SGLT1 (which transports glucose from the lumen into epithelial cells), SGLT3a does not function as a sugar transporter, at least at physiological pH.16
To investigate its function in vivo, we performed a series of phenotypic analyses using SGLT3a knockout mice. The knockout mice appeared physiologically normal under standard conditions; their food intake, body weight, and glucose tolerance were comparable to those of control littermates.
Importantly, however, maternal gut epithelial growth—both in terms of crypt-villus morphology and epithelial turnover—was significantly reduced in SGLT3a knockout mice during lactation. This effect appears to be specific to the reproductive state, as we did not observe similar phenotypes in non-pregnant female knockout mice. We also observed that progenitor proliferation was dampened in intestinal organoids—mini-guts grown in vitro—derived from SGLT3a knockout mice, suggesting a gut-specific role of SGLT3a in regulating maternal gut epithelial growth.
What are the downstream signals?
How does SGLT3a control villus growth during reproduction? We found that the population of progenitor cells expressing Fgfbp1—a known marker for isthmus progenitors17,18—was reduced in SGLT3a knockout mice. Since SGLT3a is not expressed in progenitor cells themselves, we hypothesise that SGLT3a expressed in enterocytes regulates progenitor proliferation extrinsically through Fgfbp1 expression.
Our electrophysiological experiments demonstrated that SGLT3a responds to protons and sodium, but not to sugars. Sodium is an especially intriguing candidate, as we observed that dietary sodium can mimic the effects of reproduction on the gut epithelium. Therefore, it would be interesting to further explore whether dietary sodium is essential for maternal organ remodelling, including that of the small intestine.
What are the upstream signals?
Could the upregulation of SGLT3a be driven by increased food intake during reproduction? This seems unlikely, as SGLT3a expression is already elevated by early pregnancy (gestational day 7), which precedes the marked increase in maternal food intake observed in mid-pregnancy. This suggests that other signals may be responsible for inducing SGLT3a expression in the intestinal epithelium.
One possible group of candidates is reproductive hormones. In our single-cell transcriptomic analysis of intestinal epithelial cells, we observed broad expression of the prolactin receptor, whereas estrogen and progesterone receptors were either undetectable or expressed at very low levels. Supporting this, we found that treating intestinal organoids with prolactin led to upregulation of SGLT3a expression.
These findings suggest that reproductive hormones—particularly prolactin—may play a role in this process by driving pregnancy-specific SGLT3a induction. Further research is needed to investigate how hormonal signals, or signals originating outside the intestinal epithelium,5 contribute to SGLT3a induction and maternal gut growth during reproduction.
What is the physiological relevance?
Despite no significant difference in litter size at birth, we observed a lower survival rate among pups born to SGLT3a knockout mothers compared to those born to wild-type mothers. Although we cannot exclude the possibility that this phenotype is influenced by SGLT3a function outside the gut—given that whole-body knockout mice were used—our findings suggest that SGLT3a contributes to reproductive success by sustaining maternal gut growth during pregnancy. It would be intriguing to investigate the long-term effects on both mothers and offspring, as excessive weight gain during pregnancy and after childbirth in humans is known to be associated with an increased risk of chronic diseases in mothers and future obesity in their children.
Access the article: Ameku T, Laddach A, Beckwith H, Milona A, Rogers LS, Schwayer C, Nye E, Tough IR, Thoumas JL, Gautam UK, Wang YF, Jha S, Castano-Medina A, Amourda C, Vaelli PM, Gevers S, Irvine EE, Meyer L, Andrew I, Choi KL, Patel B, Francis AJ, Studd C, Game L, Young G, Murphy KG, Owen B, Withers DJ, Rodriguez-Colman M, Cox HM, Liberali P, Schwarzer M, Leulier F, Pachnis V, Bellono NW, Miguel-Aliaga I. Growth of the maternal intestine during reproduction. Cell. 2025 Mar 19:S0092-8674(25)00200-4. doi: 10.1016/j.cell.2025.02.015. Epub ahead of print. PMID: 40112802.
References
- Napso T, Yong HEJ, Lopez-Tello J, Sferruzzi-Perri AN. The Role of Placental Hormones in Mediating Maternal Adaptations to Support Pregnancy and Lactation. Front Physiol. 2018 Aug 17;9:1091. doi: 10.3389/fphys.2018.01091. PMID: 30174608.
- Hammond KA. Adaptation of the maternal intestine during lactation. J Mammary Gland Biol Neoplasia. 1997 Jul;2(3):243-52. doi: 10.1023/a:1026332304435. PMID: 10882308.
- Speakman JR. The physiological costs of reproduction in small mammals. Philos Trans R Soc Lond B Biol Sci. 2008 Jan 27;363(1490):375-98. doi: 10.1098/rstb.2007.2145. PMID: 17686735.
- Poo LJ, Lew W, Addis T. Protein anabolism of organs and tissues during pregnancy and lactation. J. Biol. Chem. 1939 Volume 128, Issue 1, Pages 69-77, ISSN 0021-9258.
- Onji M, Sigl V, Lendl T, Novatchkova M, Ullate-Agote A, Andersson-Rolf A, Kozieradzki I, Koglgruber R, Pai TP, Lichtscheidl D, Nayak K, Zilbauer M, Carranza García NA, Sievers LK, Falk-Paulsen M, Cronin SJF, Hagelkruys A, Sawa S, Osborne LC, Rosenstiel P, Pasparakis M, Ruland J, Takayanagi H, Clevers H, Koo BK, Penninger JM. RANK drives structured intestinal epithelial expansion during pregnancy. Nature. 2025 Jan;637(8044):156-166. doi: 10.1038/s41586-024-08284-1. Epub 2024 Dec 4. PMID: 39633049.
- Blackie L, Gaspar P, Mosleh S, Lushchak O, Kong L, Jin Y, Zielinska AP, Cao B, Mineo A, Silva B, Ameku T, Lim SE, Mao Y, Prieto-Godino L, Schoborg T, Varela M, Mahadevan L, Miguel-Aliaga I. The sex of organ geometry. Nature. 2024 Jun;630(8016):392-400. doi: 10.1038/s41586-024-07463-4. Epub 2024 May 29. PMID: 38811741.
- White MA, Bonfini A, Wolfner MF, Buchon N. Drosophila melanogaster sex peptide regulates mated female midgut morphology and physiology. Proc Natl Acad Sci U S A. 2021 Jan 5;118(1):e2018112118. doi: 10.1073/pnas.2018112118. PMID: 33443193.
- Hadjieconomou D, King G, Gaspar P, Mineo A, Blackie L, Ameku T, Studd C, de Mendoza A, Diao F, White BH, Brown AEX, Plaçais PY, Préat T, Miguel-Aliaga I. Enteric neurons increase maternal food intake during reproduction. Nature. 2020 Nov;587(7834):455-459. doi: 10.1038/s41586-020-2866-8. Epub 2020 Oct 28. Erratum in: Nature. 2020 Dec;588(7839):E36. doi: 10.1038/s41586-020-3013-2. PMID: 33116314.
- Zipper L, Jassmann D, Burgmer S, Görlich B, Reiff T. Ecdysone steroid hormone remote controls intestinal stem cell fate decisions via the PPARγ-homolog Eip75B in Drosophila. Elife. 2020 Aug 10;9:e55795. doi: 10.7554/eLife.55795. PMID: 32773037.
- Ahmed SMH, Maldera JA, Krunic D, Paiva-Silva GO, Pénalva C, Teleman AA, Edgar BA. Fitness trade-offs incurred by ovary-to-gut steroid signalling in Drosophila. Nature. 2020 Aug;584(7821):415-419. doi: 10.1038/s41586-020-2462-y. Epub 2020 Jul 8. PMID: 32641829.
- Hudry B, de Goeij E, Mineo A, Gaspar P, Hadjieconomou D, Studd C, Mokochinski JB, Kramer HB, Plaçais PY, Preat T, Miguel-Aliaga I. Sex Differences in Intestinal Carbohydrate Metabolism Promote Food Intake and Sperm Maturation. Cell. 2019 Aug 8;178(4):901-918.e16. doi: 10.1016/j.cell.2019.07.029. PMID: 31398343.
- Hudry B, Khadayate S, Miguel-Aliaga I. The sexual identity of adult intestinal stem cells controls organ size and plasticity. Nature. 2016 Feb 18;530(7590):344-8. doi: 10.1038/nature16953. PMID: 26887495.
- Reiff T, Jacobson J, Cognigni P, Antonello Z, Ballesta E, Tan KJ, Yew JY, Dominguez M, Miguel-Aliaga I. Endocrine remodelling of the adult intestine sustains reproduction in Drosophila. Elife. 2015 Jul 28;4:e06930. doi: 10.7554/eLife.06930. PMID: 26216039.
- CAMPBELL RM, FELL BF. GASTRO-INTESTINAL HYPERTROPHY IN THE LACTATING RAT AND ITS RELATION TO FOOD INTAKE. J Physiol. 1964 May;171(1):90-7. doi: 10.1113/jphysiol.1964.sp007363. PMID.
- Casirola DM, Ferraris RP. Role of the small intestine in postpartum weight retention in mice. Am J Clin Nutr. 2003 Dec;78(6):1178-87. doi: 10.1093/ajcn/78.6.1178. PMID: 14668281.
- Barcelona S, Menegaz D, Díez-Sampedro A. Mouse SGLT3a generates proton-activated currents but does not transport sugar. Am J Physiol Cell Physiol. 2012 Apr 15;302(8):C1073-82. doi: 10.1152/ajpcell.00436.2011. Epub 2012 Feb 1. PMID: 22301059.
- Capdevila C, Miller J, Cheng L, Kornberg A, George JJ, Lee H, Botella T, Moon CS, Murray JW, Lam S, Calderon RI, Malagola E, Whelan G, Lin CS, Han A, Wang TC, Sims PA, Yan KS. Time-resolved fate mapping identifies the intestinal upper crypt zone as an origin of Lgr5+ crypt base columnar cells. Cell. 2024 Jun 6;187(12):3039-3055.e14. doi: 10.1016/j.cell.2024.05.001. PMID: 38848677.
- Malagola E, Vasciaveo A, Ochiai Y, Kim W, Zheng B, Zanella L, Wang ALE, Middelhoff M, Nienhüser H, Deng L, Wu F, Waterbury QT, Belin B, LaBella J, Zamechek LB, Wong MH, Li L, Guha C, Cheng CW, Yan KS, Califano A, Wang TC. Isthmus progenitor cells contribute to homeostatic cellular turnover and support regeneration following intestinal injury. Cell. 2024 Jun 6;187(12):3056-3071.e17. doi: 10.1016/j.cell.2024.05.004. PMID: 38848678.