A journey of understanding the distinct molecular mechanisms underlying plant photoperiodism
Posted by Qingqing Wang, on 29 February 2024
In a new study, Joshua Gendron and colleagues find that plants can measure two different photoperiods to independently control seasonal flowering and growth, and the vegetative growth is partially dependent on the production of myo-inositol, a precursor required in many processes that control growth (1). First author Qingqing Wang takes us through the story behind the paper.
After earning my PhD in fish reproductive physiology from a laboratory in China, I ventured to the US and secured a postdoctoral position in the Gendron lab, initiating my journey into the field of botany. While transitioning from zoology to botany presented significant hurdles, my background in biology, which encompassed genes, molecules, and proteins, eased the process of assimilating knowledge into the botanical sphere. Fortunately, the steadfast support of my lab colleagues, along with Josh Gendron’s encouragement, fostered within me the belief that creativity and problem-solving acumen are indispensable in scientific research, igniting a fervent passion for botanical pursuits.
In our laboratory, we have elucidated a metabolic daylength measurement (MDLM) system that is crucial for regulating plant growth under short winter photoperiod conditions. Notably, the post-dusk induction of one gene, PHLOEM PROTEIN 2-A13 (PP2-A13), in short days is pivotal for sustaining plant growth in winter but not summer photoperiod (2). This led me to speculate about the existence of a contrasting pathway that is regulated by MDLM system controlling plant summer growth. My exploration of photoperiod-related literature primarily uncovered research focused on the CONSTANS- FLOWERING LOCUS T (CO-FT) module’s role in regulating flowering, leaving much unknown about other pathways controlling plants’ summer growth.
MIPS1 as a model to study photoperiod-controlled growth.
Delving into the RNA-seq database curated by Gendron lab (3), I meticulously examined gene expression profiles from RNA-seq, seeking candidates exhibiting heightened expression under summer long day conditions. Amidst the constraints of Covid-induced isolation, devoid of laboratory benchwork, I seized the opportunity to immerse myself in extensive literature reviews pertaining to each intriguing gene. I came across a gene called MYOINOSITOL-1-PHOSPHATE SYNTHASE 1 (MIPS1), which plays a pivotal role in myo-inositol biosynthesis. Myo-inositol is an essential sugar that governs various cellular processes crucial for growth regulation. Notably, MIPS1 displayed elevated expression under summer long-day photoperiods, and we discovered that its mutant counterpart, mips1, exhibited specific growth defects under summer long-day conditions but not winter short-day conditions, contrasting with the pp2-a13 mutant. We observed that MIPS1 has a long-day-specific growth effect, prompting us to investigate whether growth is photoperiod-controlled and how MIPS1 responds. To address this, I cultivated both wild type and mips1 mutant plants under critical photoperiod conditions and monitored their growth. As the daytime lengthened, wild type growth was minimal in photoperiods less than 12 hours light to 12 hours dark (12L:12D). However, in photoperiods of 12L:12D or longer, wild type growth accelerated significantly, while the mips1 mutant exhibited clear growth defects. Upon seeing these results, I was excited to share the findings with everyone. The two years of effort dedicated to growing plants under various conditions in the same growth chamber proved worthwhile. These results indicate that growth is indeed controlled by photoperiod and requires MIPS1.
Those findings prompted us to investigate the potential involvement of the CO-FT mechanism in regulating MIPS1 expression. Interestingly, genetic data indicated that MIPS1 expression is not controlled by the CO-FTpathway, which led us to wonder a pivotal question: What regulatory network governs MIPS1 expression, thereby determining plant summer growth rate?
Parallel control by MDLM for MIPS1 required for long day rapid growth, and CO-FT for photoperiod flowering.
We employed a starch-less pgm mutant with a dysfunctional MDLM system to examine the regulatory effect of MDLM on MIPS1 expression. As expected, the photoperiodic expression of MIPS1 was absent in the pgm mutant, confirming our hypothesis that MDLM system regulates MIPS1 expression. Then I think about how to design an experiment to decouple photoperiodic growth and photoperiodic flowering. Exposing the plant to low light intensity, nearing the compensation point where photosynthesis equals respiration, could exhaust starch and sucrose reserves and therefore impeding MDLM but not CO-FT system. I grew plants in low light intensity condition and found that the mips1 mutant phenotype disappeared, while flowering time remained consistent with long-day conditions, which is making us exciting. This sustained our model that MDLM regulates plant growth in parallel to CO-FT regulated flowering. To further test this model, I propose a classic experiment akin to the “night break” experiments used to test photoperiodic flowering. In “night break” flowering experiments, long day plant flowers in long day but not short day photoperiod. However, introducing short pulses of light during the night in short-day conditions promotes flowering. Similarly, to assess whether photoperiod regulates rapid growth, we propose dividing the daytime in long-day conditions into two segments: one exposed to high light intensity and the other to low intensity. We found that the mips1 mutant phenotype would manifest in the segment under intense light, while the wild-type size would exceed that of the segment under light exposure during the initial portion. Moreover, flowering timing in both scenarios would mirror that of long-day conditions. This suggests that plants can discern an absolute photoperiod using low light-sensing photoreceptor mechanisms to regulate flowering time. Concurrently, they can gauge the photosynthetic duration as a metabolic day length to govern growth.
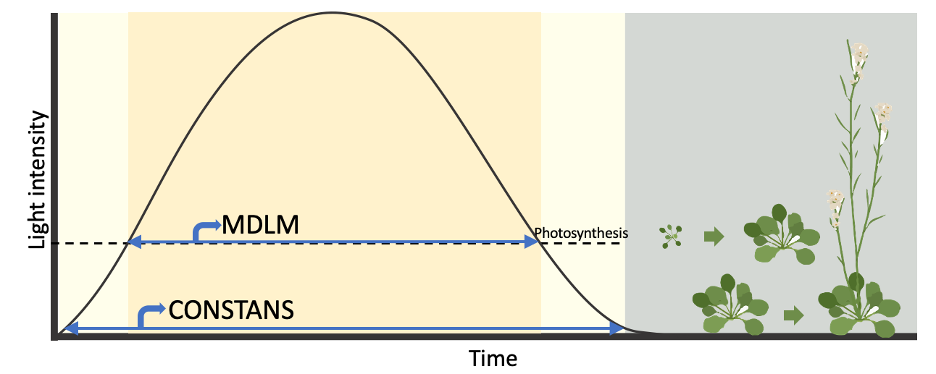
In the end…
This journey has taught me that in scientific research, one must be passionate, diligent, possess problem-solving skills, embrace teamwork, and be willing to challenge and follow classic principles. This model has filled and expanded our understanding of plant regulation by photoperiod, which extends beyond just flowering time; there are many other processes for photoperiodic regulation. Moreover, by utilizing different durations and intensities of light exposure, we can achieve separate regulation of flowering and growth in crops, leading to conservation of energy resources for crops in the future.
References
- Q. Wang, W. Liu, C. C. Leung, D. A. Tarté, J. M. Gendron, Plants distinguish different photoperiods to independently control seasonal flowering and growth. Science 383, eadg9196 (2024). https://doi.org/10.1126/science.adg9196; PMID: 38330117
- W. Liu, A Feke, C. C. Leung, D. A. Tarte´, W Yuan, M. Vanderwall, G. Sager, X. Wu, A. Schear, D. A. Clark, B. C. Thines, and J. M. Gendron, Ametabolic daylength measurement system mediates winter photoperiodism in plants. Dev. Cell 56, 2501–2515.e5(2021). doi: 10.1016/j.devcel.2021.07.016; pmid: 34407427
- C. C. Leung, D. A. Tarté, L. S. Oliver, Q. Wang, J. M. Gendron, Systematic characterization of photoperiodic gene expression patterns reveals diverse seasonal transcriptional systems in Arabidopsis. PLOS Biol. 21, e3002283 (2023). doi: 10.1371/journal.pbio.3002283; pmid: 376990558. T. C. Mockler et al., The DIURNAL project: DIURNAL
- J. M. Gendron, D. Staiger, New Horizons in Plant Photoperiodism. Annu. Rev. Plant Biol. 74, 481–509 (2023). doi: 10.1146/annurev-arplant-070522-055628; pmid: 36854481
- J. M. Gendron, C. C. Leung, W. Liu, Energy as a seasonal signal for growth and reproduction. Curr. Opin. Plant Biol. 63, 102092(2021). doi: 10.1016/j.pbi.2021.102092; pmid: 34461431