Genetically modified non-human primate fetuses as models to study the role of candidate genes for increasing size and folding of the human neocortex during development and evolution
Posted by Michael Heide, on 27 July 2020
By Michael Heide and Wieland B. Huttner
Introduction
The neocortex is the seat of our higher cognitive abilities that distinguish us from other mammals and that make us human (Rakic, 2009). One basis for this crucial feature is the increase in the size of the neocortex during hominin evolution, culminating in modern humans (Striedter, 2005, Azevedo et al., 2009, Kaas, 2013, Sousa et al., 2017, Molnar et al., 2019). A key issue in this context is the identification of genomic determinants that underlie the increased growth of the neocortex during brain development. Once candidate genes have been identified, the challenge then is to demonstrate their role in neocortex expansion in an appropriate system. Here, we summarize the background information that suggested the human-specific gene ARHGAP11B to be a candidate to have contributed to neocortex expansion and folding during human evolution, and explain the rationale for demonstrating this role in an ARHGAP11B-transgenic non-human primate model system, that is, fetuses of the common marmoset. Such functional, developmental and evolutionary studies have so far been rarely performed in transgenic non-human primates. In our recent publication, Heide et al. 2020, we show that the human-specific gene ARHGAP11B, when expressed to physiological levels in the fetal marmoset neocortex, indeed causes neocortex expansion and increases neocortex folding, implying that this gene significantly contributed to human neocortex evolution (Heide et al., 2020). Moreover, we hope that our findings provide evidence in support of the usefulness and feasibility of genetically modified non-human primates for neurodevelopmental and evolutionary studies, as well as other fields of neurobiology.
A need for genetically modifiable non-human primate models in neurobiological studies
Which systems are most appropriate for studying the development of the neocortex with the aim to gain insight into the development and evolution of the human neocortex? Commonly used animal models that lend themselves to genetic modifications have been smooth-brained (lissencephalic) rodents (mouse, rat) and the ferret, a carnivore with a folded (gyrencephalic) brain (Molnar et al., 2006, Sun and Hevner, 2014, Kawasaki, 2018, Zhao and Bhattacharyya, 2018). However, many findings about neocortical development obtained in these model systems cannot be translated as such to humans, as is exemplified, in particular, in the case of many mouse disease models (e.g., mouse models of primary microcephaly (Pinson et al., 2019)).
A recently emerged, promising in vitro model system of human neocortical development that overcomes some of these limitations are human brain organoids (Kadoshima et al., 2013, Lancaster et al., 2013, Camp et al., 2015, Qian et al., 2016, Quadrato et al., 2017). These are 3D cellular assemblies that model the tissue of certain brain regions over a particular developmental time window and recapitulate many aspects of the cytoarchitecture and cell-type complexity of the modelled brain tissue (Heide et al., 2018). Moreover, comparison of brain organoids of human vs. non-human primate origin (macaque, chimpanzee, orang-utan) has led to the identification of human-specific features of neocortical development (Otani et al., 2016, Mora-Bermudez et al., 2016, Pollen et al., 2019, Kanton et al., 2019). Importantly, brain organoids can easily be genetically modified (Fischer et al., 2019), and human brain organoids have been shown to recapitulate gene expression patterns of fetal human neocortex (Camp et al., 2015). However, despite its promise, the brain organoid model system still has certain limitations, such that is does not fully recapitulate the cytoarchitecture, cell-type composition and late and postnatal stages of neocortex development (Heide et al., 2018). Moreover, cell-type specification in brain organoids seems to be impaired due to cellular stress (Bhaduri et al., 2020).
Hence, fetal human brain tissue ex vivo (Rakic, 2006, Florio et al., 2015, Johnson et al., 2015, Pollen et al., 2015, Kalebic et al., 2019, Namba et al., 2020) and non-human primate models (Smart et al., 2002, Rakic, 2006, Kelava et al., 2012, Garcia-Moreno et al., 2012, Betizeau et al., 2013) have been increasingly used to overcome the limitations of the rodent and ferret models and of brain organoids for studying the development of the neocortex with the aim to gain insight into the development and evolution of the human neocortex. However, the use of fetal human brain tissue ex vivo is limited to early stages of neocortical development, and genetic modification approaches can only be conducted – for obvious ethical reasons – with ex vivo fetal human brain tissue. It is for these reasons that genetically modifiable non-human primate models have recently emerged as system of choice to gain insight into human neocortex development and evolution (Sasaki et al., 2009, Niu et al., 2010, Niu et al., 2014, Shi et al., 2019, Heide et al., 2020). A major advantage of non-human primate models is that in terms of morphology, cell-type composition, gene expression and interaction partners, these models are much closer to human neocortex development than the rodent and ferret models. Moreover, genetically modifiable non-human primate models are likely to have huge potential in generating models of neurological and neurodevelopmental disorders.
ARHGAP11B — a human-specific gene that may drive human neocortex expansion and folding?
The ARHGAP11B gene evolved ~5 mya, that is, after the split of the lineage leading to modern humans and the lineage leading to chimpanzee and bonobo, by segmental duplication of the widespread gene ARHGAP11A (Sudmant et al., 2010). In other words, ARHGAP11B is only found in humans and hence is a human-specific gene. However, ARHGAP11B is not a simple copy of ARHGAP11A, as the ARHGAP11B protein corresponds to only the N-terminal one quarter of the ARHGAP11A protein and, importantly, possesses a unique, human-specific 47 amino acid sequence in its C-terminal domain that is key for its function (Florio et al., 2015, Namba et al., 2020). This unique C-terminal protein sequence arises from a single C-to-G nucleotide substitution in ARHGAP11B that generated a novel splice donor site, resulting in the loss of 55 nucleotides upon mRNA splicing. This loss in turn causes a shift in the reading frame, leading to ARHGAP11B’s unique C-terminal 47 amino acid sequence (Florio et al., 2016). Previous functional analyses by strong overexpression of this gene in embryonic mouse (Florio et al., 2015) and ferret (Kalebic et al., 2018) neocortex showed increased numbers of basal progenitor cells and of upper-layer neurons, the class of progenitors and type of cortical neurons, respectively, that have been implicated in neocortex expansion (Lui et al., 2011, Fame et al., 2011, Borrell and Reillo, 2012, Betizeau et al., 2013, Florio and Huttner, 2014, Dehay et al., 2015, Lodato and Arlotta, 2015). Moreover, in the case of mouse, ARHGAP11B overexpression could induce folding of the normally smooth neocortex (Florio et al., 2015). These findings suggested that ARHGAP11B is a candidate gene to have contributed to neocortex expansion and folding during human evolution. However, the key question was whether this gene, when expressed to physiological levels and in a primate model that is evolutionarily closer to human than mouse or ferret, would increase neocortex size and folding.
Which genetically modifiable non-human primate model to choose?
We therefore searched for a genetically modifiable primate model that is evolutionarily as close as (ethically) possible to human and that possesses all relevant stem cell populations at the correct relative abundance in the developing neocortex, to allow us to explore the potential role of ARHGAP11B in the expansion of the neocortex during development and human evolution. This confined our choice to two non-human primate models, the common marmoset (Callithrix jacchus) and the macaque (eitherMacaca mulatta or Macaca fascicularis). We chose to focus on the marmoset, as this New World monkey, while exhibiting many of the features of the large and folded human neocortex, has – in contrast to the macaque – a small and unfolded neocortex, making it thus an ideal model to study ARHGAP11B’s potential contribution to neocortical expansion and folding.
ARHGAP11B increases fetal primate neocortex size and folding
In Heide et al. 2020, we generated, in collaboration with the research groups of Erika Sasaki and Hideyuki Okano in Japan who have pioneered the transgenic marmoset technology (Sasaki et al., 2009), ARHGAP11B-transgenic marmoset fetuses (Figure A) that expressed ARHGAP11B in the developing neocortex under the control of its own, human promoter (Heide et al., 2020).
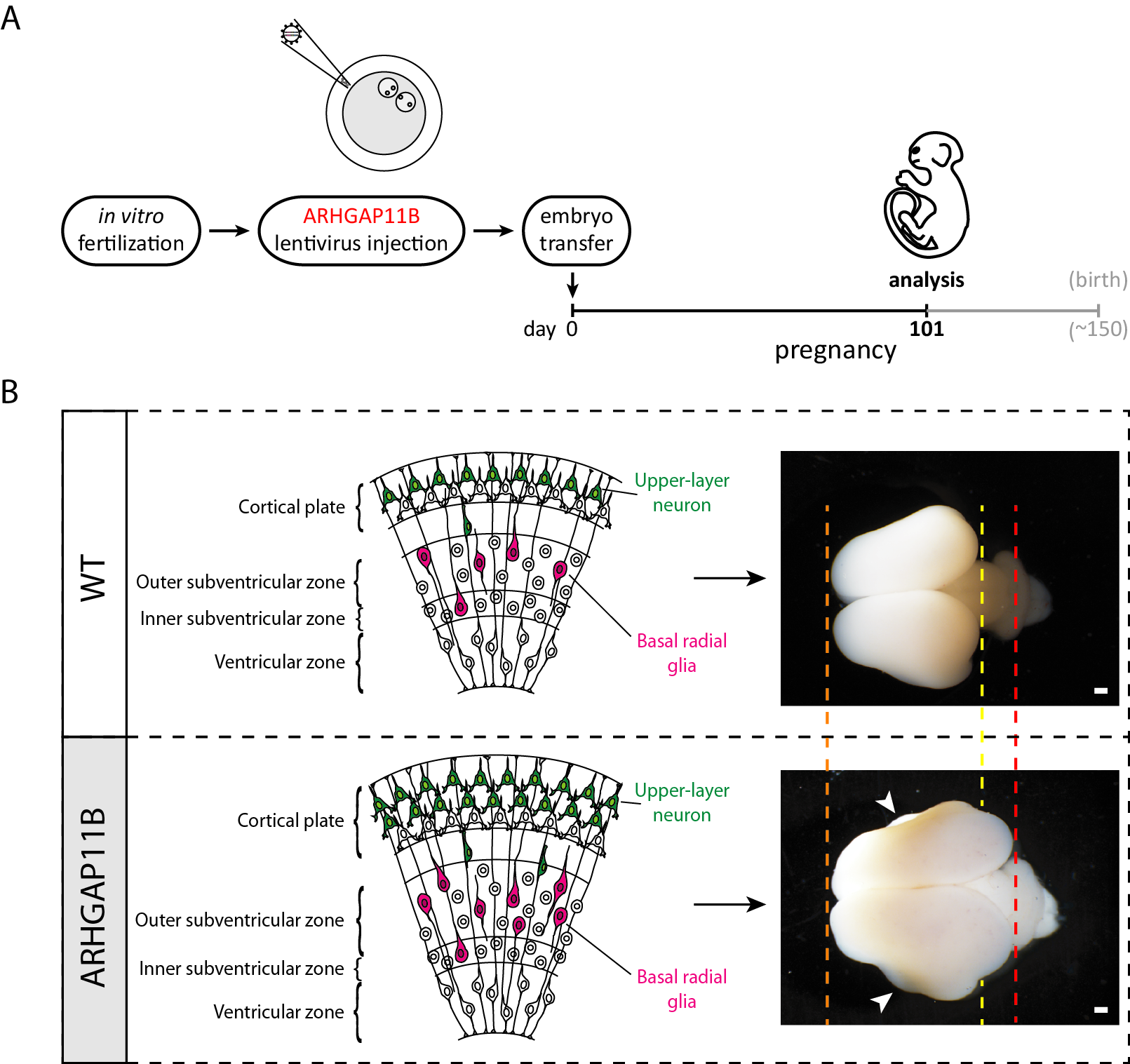
In the neocortex of these ARHGAP11B-transgenic marmoset fetuses, ARHGAP11B was expressed to physiological levels, that is, to similar levels as in fetal human neocortex, and its expression within the neocortex was confined to neural stem and progenitor cells (Heide et al., 2020). Furthermore, this ARHGAP11B expression increased the abundance of basal radial glia (Heide et al., 2020) – the basal progenitor cell type thought to play a key role in neocortex expansion and folding, notably in human neocortex development (Lui et al., 2011, Borrell and Reillo, 2012 Florio and Huttner, 2014). This was accompanied by an increase in upper-layer neurons (Heide et al., 2020) — the cortical neuron type thought to strongly contribute to our higher cognitive abilities (Fame et al., 2011). At the supracellular level, this ARHGAP11B expression resulted in increased neocortex size and induced folding of the normally unfolded fetal marmoset neocortex (Heide et al., 2020) (Figure B). In summary, our study strongly suggests that ARHGAP11B did contribute to neocortex expansion and folding in the course of human evolution.
Ethical considerations
Experiments involving non-human primates have been under constant public and scientific debate, and it is perfectly legitimate to scrutinize whether or not such experiments are really necessary and to ask whether other animal model systems are available that are appropriate to address the same scientific questions. Furthermore, experiments involving non-human primates should be performed according to the highest ethical standards.
In our case, we reasoned that in order to answer the question whether the human-specific gene ARHGAP11B contributed to neocortex expansion and folding during human evolution, it was necessary to express ARHGAP11B in a suitable non-human primate model, that is, the common marmoset. We limited these experiments and analyses to fetal stages, for two main reasons. First, should ARHGAP11Bexpression increase neocortex size and neuron numbers as we anticipated, the consequences for the postnatal and adult marmosets with regard to behaviour and nervous system performance might be unpredictable. In this context, one should realize that increases in neocortex size and neuron numbers are not necessarily beneficial but can be the underlying cause of certain human diseases (e.g., megalencephaly, polymicrogyria), and accordingly could lead to suffering of the animals (e.g., from seizures). To avoid generating ARHGAP11B-expressing postnatal and adult marmosets, all ARHGAP11B-expressing marmoset fetuses analyzed in our study were newly and individually generated (rather than being descendants of an ARHGAP11B-expressing marmoset line). Second, our previous data had indicated that ARHGAP11B expression increased basal progenitors and upper-layer neurons during the development of the neocortex. This suggested that in our case an analysis of fetal stages of ARHGAP11B-expressing marmosets would be appropriate and constructive, and would allow closely monitoring neocortex development and analyzing it at the relevant stages.
We hope that our study shows that genetically modified non-human primate fetuses can be superior models to understand human neocortex development and evolution. Furthermore, our work will hopefully encourage other researchers from the present and other fields of neurobiology (including disease modelling) to study genetically modified non-human primate models in the future, as (i) such experiments can be performed in an ethically justifiable way (see above), (ii) the results may answer questions that other, non-primate models cannot answer, and (iii) the results most likely can be directly translated to humans.
References
AZEVEDO, F. A. C., CARVALHO, L. R. B., GRINBERG, L. T., FARFEL, J. M., FERRETTI, R. E. L., LEITE, R. E. P., JACOB, W., LENT, R. & HERCULANO-HOUZEL, S. 2009. Equal numbers of neuronal and nonneuronal cells make the human brain an isometrically scaled-up primate brain. J. Comp. Neurol. , 513, 532-541.
BETIZEAU, M., CORTAY, V., PATTI, D., PFISTER, S., GAUTIER, E., BELLEMIN-MÉNARD, A., AFANASSIEFF, M., HUISSOUD, C., DOUGLAS, R. J., KENNEDY, H. & DEHAY, C. 2013. Precursor diversity and complexity of lineage relationships in the outer subventricular zone of the primate. Neuron, 80, 442-457.
BHADURI, A., ANDREWS, M. G., MANCIA LEON, W., JUNG, D., SHIN, D., ALLEN, D., JUNG, D., SCHMUNK, G., HAEUSSLER, M., SALMA, J., POLLEN, A. A., NOWAKOWSKI, T. J. & KRIEGSTEIN, A. R. 2020. Cell stress in cortical organoids impairs molecular subtype specification. Nature, 578, 142-148.
BORRELL, V. & REILLO, I. 2012. Emerging roles of neural stem cells in cerebral cortex development and evolution. Dev Neurobiol, 72, 955-971.
CAMP, J. G., BADSHA, F., FLORIO, M., KANTON, S., GERBER, T., WILSCH-BRÄUNINGER, M., LEWITUS, E., SYKES, A., HEVERS, W., LANCASTER, M., KNOBLICH, J. A., LACHMANN, R., PÄÄBO, S., HUTTNER, W. B. & TREUTLEIN, B. 2015. Human cerebral organoids recapitulate gene expression programs of fetal neocortex development. Proc Natl Acad Sci U S A, 112,15672-7.
DEHAY, C., KENNEDY, H. & KOSIK, K. S. 2015. The outer subventricular zone and primate-specific cortical complexification. Neuron, 85, 683-694.
FAME, R. M., MACDONALD, J. L. & MACKLIS, J. D. 2011. Development, specification, and diversity of callosal projection neurons. Trends Neurosci, 34, 41-50.
FISCHER, J., HEIDE, M. & HUTTNER, W. B. 2019. Genetic modification of brain organoids. Front Cell Neurosci, 13, 558.
FLORIO, M., ALBERT, M., TAVERNA, E., NAMBA, T., BRANDL, H., LEWITUS, E., HAFFNER, C., SYKES, A., WONG, F. K., PETERS, J., GUHR, E., KLEMROTH, S., PRUFER, K., KELSO, J., NAUMANN, R., NÜSSLEIN, I., DAHL, A., LACHMANN, R., PÄÄBO, S. & HUTTNER, W. B. 2015. Human-specific gene ARHGAP11B promotes basal progenitor amplification and neocortex expansion. Science, 347, 1465-1470.
FLORIO, M. & HUTTNER, W. B. 2014. Neural progenitors, neurogenesis and the evolution of the neocortex. Development, 141, 2182-2194.
FLORIO, M., NAMBA, T., PÄÄBO, S., HILLER, M. & HUTTNER, W. B. 2016. A single splice site mutation in human-specific ARHGAP11B causes basal progenitor amplification. Sci Adv, 2,e1601941.
GARCIA-MORENO, F., VASISTHA, N. A., TREVIA, N., BOURNE, J. A. & MOLNAR, Z. 2012. Compartmentalization of cerebral cortical germinal zones in a lissencephalic primate and gyrencephalic rodent. Cereb. Cortex, 22, 482-492.
HEIDE, M., HAFFNER, C., MURAYAMA, A., KUROTAKI, Y., SHINOHARA, H., OKANO, H., SASAKI, E. & HUTTNER, W. B. 2020. Human-specific ARHGAP11B increases size and folding of primate neocortex in the fetal marmoset. Science, eabb2401 [published online ahead of print].
HEIDE, M., HUTTNER, W. B. & MORA-BERMUDEZ, F. 2018. Brain organoids as models to study human neocortex development and evolution. Curr Opin Cell Biol, 55, 8-16.
JOHNSON, M. B., WANG, P. P., ATABAY, K. D., MURPHY, E. A., DOAN, R. N., HECHT, J. L. & WALSH, C. A. 2015. Single-cell analysis reveals transcriptional heterogeneity of neural progenitors in human cortex. Nat. Neurosci., 18, 637-646.
KAAS, J. H. 2013. The evolution of brains from early mammals to humans. WIREs Cogn. Sci., 4, 33-45.
KADOSHIMA, T., SAKAGUCHI, H., NAKANO, T., SOEN, M., ANDO, S., EIRAKU, M. & SASAI, Y. 2013. Self-organization of axial polarity, inside-out layer pattern, and species-specific progenitor dynamics in human ES cell-derived neocortex. Proc Natl Acad Sci U S A, 110, 20284-9.
KALEBIC, N., GILARDI, C., ALBERT, M., NAMBA, T., LONG, K. R., KOSTIC, M., LANGEN, B. & HUTTNER, W. B. 2018. Human-specific ARHGAP11B induces hallmarks of neocortical expansion in developing ferret neocortex. eLife, 7, e41241.
KALEBIC, N., GILARDI, C., STEPIEN, B., WILSCH-BRÄUNINGER, M., LONG, K. R., NAMBA, T., FLORIO, M., LANGEN, B., LOMBARDOT, B., SHEVCHENKO, A., KILIMANN, M. W., KAWASAKI, H., WIMBERGER, P. & HUTTNER, W. B. 2019. Neocortical expansion due to increased proliferation of basal progenitors is linked to changes in their morphology. Cell Stem Cell, 24, 535-550 e9.
KANTON, S., BOYLE, M. J., HE, Z. S., SANTEL, M., WEIGERT, A., SANCHIS-CALLEJA, F., GUIJARRO, P., SIDOW, L., FLECK, J. S., HAN, D. D., QIAN, Z. Z., HEIDE, M., HUTTNER, W. B., KHAITOVICH, P., PÄÄBO, S., TREUTLEIN, B. & CAMP, J. G. 2019. Organoid single-cell genomic atlas uncovers human-specific features of brain development. Nature, 574, 418-422.
KAWASAKI, H. 2018. Molecular investigations of the development and diseases of cerebral cortex folding using gyrencephalic mammal ferrets. Biol Pharm Bull, 41, 1324-1329.
KELAVA, I., REILLO, I., MURAYAMA, A. Y., KALINKA, A. T., STENZEL, D., TOMANCAK, P., MATSUZAKI, F., LEBRAND, C., SASAKI, E., SCHWAMBORN, J. C., OKANO, H., HUTTNER, W. B. & BORRELL, V. 2012. Abundant occurrence of basal radial glia in the subventricular zone of embryonic neocortex of a lissencephalic primate, the common marmoset Callithrix jacchus. Cereb. Cortex, 22, 469-481.
LANCASTER, M. A., RENNER, M., MARTIN, C. A., WENZEL, D., BICKNELL, L. S., HURLES, M. E., HOMFRAY, T., PENNINGER, J. M., JACKSON, A. P. & KNOBLICH, J. A. 2013. Cerebral organoids model human brain development and microcephaly. Nature, 501, 373-9.
LODATO, S. & ARLOTTA, P. 2015. Generating neuronal diversity in the mammalian cerebral cortex. Annu. Rev. Cell Dev. Biol., 31, 699-720.
LUI, J. H., HANSEN, D. V. & KRIEGSTEIN, A. R. 2011. Development and evolution of the human neocortex. Cell, 146, 18-36.
MOLNAR, Z., CLOWRY, G. J., SESTAN, N., ALZU’BI, A., BAKKEN, T., HEVNER, R. F., HUPPI, P. S., KOSTOVIC, I., RAKIC, P., ANTON, E. S., EDWARDS, D., GARCEZ, P., HOERDER-SUABEDISSEN, A. & KRIEGSTEIN, A. 2019. New insights into the development of the human cerebral cortex. J Anat, 235, 432-451.
MOLNAR, Z., METIN, C., STOYKOVA, A., TARABYKIN, V., PRICE, D. J., FRANCIS, F., MEYER, G., DEHAY, C. & KENNEDY, H. 2006. Comparative aspects of cerebral cortical development. Eur J Neurosci, 23, 921-34.
MORA-BERMUDEZ, F., BADSHA, F., KANTON, S., CAMP, J. G., VERNOT, B., KOHLER, K., VOIGT, B., OKITA, K., MARICIC, T., HE, Z., LACHMANN, R., PÄÄBO, S., TREUTLEIN, B. & HUTTNER, W. B. 2016. Differences and similarities between human and chimpanzee neural progenitors during cerebral cortex development. eLife, 5, e18683.
NAMBA, T., DOCZI, J., PINSON, A., XING, L., KALEBIC, N., WILSCH-BRÄUNINGER, M., LONG, K. R., VAID, S., LAUER, J., BOGDANOVA, A., BORGONOVO, B., SHEVCHENKO, A., KELLER, P., DRECHSEL, D., KURZCHALIA, T., WIMBERGER, P., CHINOPOULOS, C. & HUTTNER, W. B. 2020. Human-specific ARHGAP11B acts in mitochondria to expand neocortical progenitors by glutaminolysis. Neuron, 105, 867-881 e9.
NIU, Y., YU, Y., BERNAT, A., YANG, S., HE, X., GUO, X., CHEN, D., CHEN, Y., JI, S., SI, W., LV, Y., TAN, T., WEI, Q., WANG, H., SHI, L., GUAN, J., ZHU, X., AFANASSIEFF, M., SAVATIER, P., ZHANG, K., ZHOU, Q. & JI, W. 2010. Transgenic rhesus monkeys produced by gene transfer into early-cleavage-stage embryos using a simian immunodeficiency virus-based vector. Proc Natl Acad Sci U S A, 107, 17663-7.
NIU, Y. Y., SHEN, B., CUI, Y. Q., CHEN, Y. C., WANG, J. Y., WANG, L., KANG, Y., ZHAO, X. Y., SI, W., LI, W., XIANG, A. P., ZHOU, J. K., GUO, X. J., BI, Y., SI, C. Y., HU, B., DONG, G. Y., WANG, H., ZHOU, Z. M., LI, T. Q., TAN, T., PU, X. Q., WANG, F., JI, S. H., ZHOU, Q., HUANG, X. X., JI, W. Z. & SHA, J. H. 2014. Generation of gene-modified cynomolgus monkey via Cas9/RNA-mediated gene targeting in one-cell embryos. Cell, 156, 836-843.
OTANI, T., MARCHETTO, M. C., GAGE, F. H., SIMONS, B. D. & LIVESEY, F. J. 2016. 2D and 3D stem cell models of primate cortical development identify species-specific differences in progenitor behavior contributing to brain size. Cell Stem Cell, 18, 467-80.
PINSON, A., NAMBA, T. & HUTTNER, W. B. 2019. Malformations of human neocortex in development – their progenitor cell basis and experimental model systems. Front Cell Neurosci, 13, 305.
POLLEN, A. A., BHADURI, A., ANDREWS, M. G., NOWAKOWSKI, T. J., MEYERSON, O. S., MOSTAJO-RADJI, M. A., DI LULLO, E., ALVARADO, B., BEDOLLI, M., DOUGHERTY, M. L., FIDDES, I. T., KRONENBERG, Z. N., SHUGA, J., LEYRAT, A. A., WEST, J. A., BERSHTEYN, M., LOWE, C. B., PAVLOVIC, B. J., SALAMA, S. R., HAUSSLER, D., EICHLER, E. E. & KRIEGSTEIN, A. R. 2019. Establishing cerebral organoids as models of human-specific brain evolution. Cell, 176, 743-756.
POLLEN, A. A., NOWAKOWSKI, T. J., CHEN, J., RETALLACK, H., SANDOVAL-ESPINOSA, C., NICHOLAS, C. R., SHUGA, J., LIU, S. J., OLDHAM, M. C., DIAZ, A., LIM, D. A., LEYRAT, A. A., WEST, J. A. & KRIEGSTEIN, A. R. 2015. Molecular identity of human outer radial glia during cortical development. Cell, 163, 55-67.
QIAN, X., NGUYEN, H. N., SONG, M. M., HADIONO, C., OGDEN, S. C., HAMMACK, C., YAO, B., HAMERSKY, G. R., JACOB, F., ZHONG, C., YOON, K. J., JEANG, W., LIN, L., LI, Y., THAKOR, J., BERG, D. A., ZHANG, C., KANG, E., CHICKERING, M., NAUEN, D., HO, C. Y., WEN, Z., CHRISTIAN, K. M., SHI, P. Y., MAHER, B. J., WU, H., JIN, P., TANG, H., SONG, H. & MING, G. L. 2016. Brain-region-specific organoids using mini-bioreactors for modeling ZIKV exposure. Cell,165, 1238-1254.
QUADRATO, G., NGUYEN, T., MACOSKO, E. Z., SHERWOOD, J. L., MIN YANG, S., BERGER, D. R., MARIA, N., SCHOLVIN, J., GOLDMAN, M., KINNEY, J. P., BOYDEN, E. S., LICHTMAN, J. W., WILLIAMS, Z. M., MCCARROLL, S. A. & ARLOTTA, P. 2017. Cell diversity and network dynamics in photosensitive human brain organoids. Nature, 545, 48-53.
RAKIC, P. 2006. A century of progress in corticoneurogenesis: from silver impregnation to genetic engineering. Cereb Cortex, 16 Suppl 1, i3-17.
RAKIC, P. 2009. Evolution of the neocortex: a perspective from developmental biology. Nat. Rev. Neurosci., 10, 724-735.
SASAKI, E., SUEMIZU, H., SHIMADA, A., HANAZAWA, K., OIWA, R., KAMIOKA, M., TOMIOKA, I., SOTOMARU, Y., HIRAKAWA, R., ETO, T., SHIOZAWA, S., MAEDA, T., ITO, M., ITO, R., KITO, C., YAGIHASHI, C., KAWAI, K., MIYOSHI, H., TANIOKA, Y., TAMAOKI, N., HABU, S., OKANO, H. & NOMURA, T. 2009. Generation of transgenic non-human primates with germline transmission. Nature, 459, 523-7.
SHI, L., LUO, X., JIANG, J., CHEN, Y., LIU, C., HU, T., LI, M., LIN, Q., LI, Y., HUANG, J., WANG, H., NIU, Y., SHI, Y., STYNER, M., WANG, J., LU, Y., SUN, X., YU, H., JI, W. & SU, B. 2019. Transgenic rhesus monkeys carrying the human MCPH1 gene copies show human-like neoteny of brain development. National Science Review, 6, 480-493.
SMART, I. H., DEHAY, C., GIROUD, P., BERLAND, M. & KENNEDY, H. 2002. Unique morphological features of the proliferative zones and postmitotic compartments of the neural epithelium giving rise to striate and extrastriate cortex in the monkey. Cereb. Cortex, 12, 37-53.
SOUSA, A. M. M., MEYER, K. A., SANTPERE, G., GULDEN, F. O. & SESTAN, N. 2017. Evolution of the human nervous system function, structure, and development. Cell, 170, 226-247.
STRIEDTER, G. F. 2005. Principles of Brain Evolution, Sinauer Associates Inc.
SUDMANT, P. H., KITZMAN, J. O., ANTONACCI, F., ALKAN, C., MALIG, M., TSALENKO, A., SAMPAS, N., BRUHN, L., SHENDURE, J. & EICHLER, E. E. 2010. Diversity of human copy number variation and multicopy genes. Science, 330, 641-646.
SUN, T. & HEVNER, R. F. 2014. Growth and folding of the mammalian cerebral cortex: from molecules to malformations. Nat. Rev. Neurosci., 15, 217-232.
ZHAO, X. & BHATTACHARYYA, A. 2018. Human models are needed for studying human neurodevelopmental disorders. Am J Hum Genet, 103, 829-857.