How we found a switch from genitalia to limbs
Posted by Anastasiia Lozovska, on 28 June 2024
While the vertebrate body consists of diverse structures formed during embryogenesis, there is a limited number of genetic regulatory modules that are repurposed in different developmental contexts. For example, the same gene, or group of genes, often play different roles in development of different embryonic structures. That is the case for the hindlimbs and external genitalia – appendages that share many developmental mechanisms and regulatory genes. In our recently published paper, we show that in the absence of Tgfbr1, precursor of external genitalia changes its response to common regulatory signals from genital-specific to limb-specific and adopts limb fate.
Tgf-beta signaling regulates the trunk to tail transition
When I started my PhD project I wanted to study the development of the main body axis. The vertebrate body consists of three main compartments: head, trunk and tail. During embryogenesis these compartments form sequentially, and together with timely transitions between them outline the body plan of an adult organism. I was particularly interested in the trunk to tail transition.
It has long been known that Growth Differentiation Factor 11 (Gdf11) is a key regulator of the trunk to tail transition [1]. In Gdf11 mutant embryos this transition is delayed, resulting in extended trunk length. However, the exact mechanism underlying Gdf11 activity remains unclear. Gdf11 is a signaling molecule of the transforming growth factor beta (Tgf-beta) superfamily and its activity regulating the trunk to tail transition is mediated by binding to a complex of membrane receptors. The partial redundancy with other Tgf-beta ligands has complicated studying molecular mechanism behind Gdf11 [2]. We though that removing one of the membrane receptors could solve this redundancy problem and help us understand genetic regulation of the transition. Previous studies have shown that Transforming growth factor beta receptor 1 (Tgfbr1) mediates Gdf11 activity in the context of trunk to tail transition, so we targeted this receptor and created a mutant mouse line [3], [4].
From the main body axis to appendages
This model helped us uncover many aspects of Tgf-beta regulation of the trunk to tail transition. Normally, during this transition, the embryo stops producing mesoderm associated with the development of internal organs and the body wall and induces caudal body appendages, such as hindlimbs and external genitalia. We showed that Tgfbr1 knock out embryos fail in all these processes [5]. However, due to Tgfbr1’s involvement in heart development and angiogenesis, which results in midgestational lethality, we could only evaluate the early phenotype in Tgfbr1 mutants [6]. To study the effects of Tgfbr1 deficiency at later developmental stages we designed another mouse model where this gene is inactivated after its requirement for heart development. We induced Tgfbr1 deletion in the embryo by administering tamoxifen to pregnant females.
Obtaining late-stage mutant embryos required crossing mice with compound genotypes and optimizing tamoxifen delivery. After months of adjustments in the protocol, we observed the first embryo with a distinct phenotype. It was very fragile and had multiple malformations in the body wall and neural tube, but the most striking feature was the duplication of the hindlimbs. When I showed the mutant to Moises, my supervisor, he said it was one of the most striking phenotypes that he had ever seen in his lab. Of course, we were curious to know how knocking out the Tgf-beta receptor led to the formation of additional hindlimbs. By then, I was already two years into my PhD, but the excitement around this finding made us pivot the project, and I had to learn a lot about limb development.
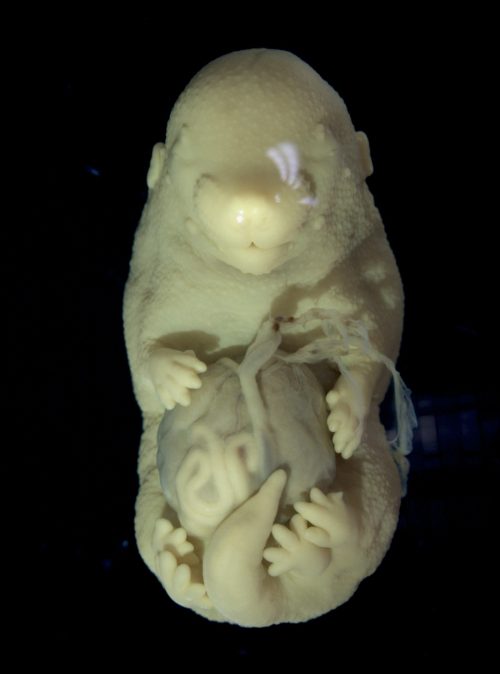
Limbs and genitalia share more than we think
To understand the phenotype first we characterized the expression patterns of limb regulatory genes at midgestational stages during early limb bud development. Interestingly, we observed that the hindlimb field in the mutants was extended posteriorly, almost reaching the genital area. The genital primordium, in turn, was underdeveloped in the mutants. This reduction in genital growth could either coincide with hindlimb duplication due to independent regulation of the two structures by Tgfbr1, or alternatively, result from the recruitment of the genital primordium into the limb field, resulting in its development into an additional set of hindlimbs. Hindlimbs and external genitalia precursors are both induced at the trunk to tail transition and, despite developing into morphologically very different appendages, share many regulatory genes [7], [8]. The commonalities between hindlimb and genitalia development prompted us to explore the latter hypothesis. We tried to recreate the activation of limb genes in the pericloacal mesenchyme (the precursor of external genitalia) by overexpression. After generating several transgenics expressing early limb specific genes in the pericloacal mesenchyme, we found that the misexpression of a single gene was insufficient to recapitulate mutant phenotype. We decided to change our approach and focus on the general mechanisms of tissue response. Given that hindlimbs and external genitalia share the expression of so many genes, how are their developmental outcomes so different?
Tgfbr1 guides tissue response by acting on chromatin state
To answer this question, we decided to look at the cis-regulatory regions in the two structures in wild type embryos and Tgfbr1 conditional knock out (cKO). The simplest way to evaluate the activity of the chromatin regions is determining whether they are compacted into nucleosome (inactive) or are nucleosome-free (accessible for transcription factors). We used ATAC-seq, which only generates sequences of open, nucleosome-free chromatin regions [9]. This analysis identified a set of genital-specific regulatory regions that lost accessibility in mutant tissue collected from genital area. The loss of accessibility in these regions could contribute to the inability to activate expression of genes required for genital growth, despite the presence of upstream transcription factors. Further analysis of the mutant extra hindlimb showed that it shared more features with genital samples than with wild type limbs, in line with the hypothesis of its genital origin. Despite that, some chromatin regions in the extra hindlimb acquired limb-type patterns. We believe that gained accessibility in the limb specific regulatory regions of the mutant genital area contributed to its development into an ectopic limb.
Of course, not every nucleosome-free chromatin region is a regulatory region. To validate our findings, we tested several potential regulatory regions using a transgenic reporter assay. To narrow the search, we focused on regions near genes known to be involved in development of limbs and external genitalia. Another criterion for identifying potential regulatory regions was evolutionary conservation. In that way, we identified several regions that drive reporter expression in the genital tubercle (GT) among those that lost accessibility in mutant genital area.
One of the most interesting changes in chromatin was found in a well characterized enhancer driving Gremlin expression in the limb. Gremlin, a secreted Bmp inhibitor, is expressed in response to Sonic Hedgehog (Shh) [10]. Both genes are required for proper limb development [11], [12]. Shh is also a driver of genital growth [13]. However, Gremlin is not expressed in the developing genitalia, despite the presence of Shh signaling. Our results indicate that in wild type genitalia, Gremlin enhancers are inaccessible to Shh regulatory activity. In contrast, in Tgfbr1 cKO embryos, one of the Gremlin enhancers is accessible, leading to ectopic Gremlin activation in the pericloacal mesenchyme. Another regulatory region that we examined in more detail was associated with GT growth and contained binding sequences for the Wnt downstream transcription factor Lef1. By generating sequential deletions of Lef1 binding sequences, we showed that reducing their number decreases reporter activity in the GT. These results illustrate how Tgfbr1 modulates response of limb and genital precursor tissues to common regulatory factors, particularly signaling pathways. This explains how loss of Tgfbr1 leads to a systemic shift in pericloacal mesenchyme response, uncovering its potential to form limbs.
Concluding remarks
The interesting phenotype of our mutant, and (I hope) the novelty of the mechanism we discovered brought a lot of attention to our preprint. Although the manuscript was rejected by two journals, it finally found its place in Nature Communications, which was immensely gratifying. I am very grateful to the reviewers for their comments and suggestions that helped us improve the manuscript.
Unexpectedly for me, the published work received a lot of media attention, likely due to the extraordinary phenotype of the Tgfbr1 cKO. While it was exciting to see my work getting attention, it made me think about the importance of science communication, especially when working with animal models, and even more when conducting basic research. The lack of direct medical application in our research made some people question the justification of our work. This experience underscored the need for clear and effective communication to convey the value and purpose of scientific research to the public.
References
[1] A. C. Mcpherron, A. M. Lawler, and S. Lee, ‘Regulation of anterior / posterior patterning of the axial skeleton by growth / differentiation factor 11’, Nature, vol. 22, no. july, pp. 1–5, 1999.
[2] A. C. McPherron, T. V. Huynh, and S. J. Lee, ‘Redundancy of myostatin and growth/differentiation factor 11 function’, BMC Dev. Biol., vol. 9, no. 1, pp. 1–9, 2009, doi: 10.1186/1471-213X-9-24.
[3] O. Andersson, E. Reissmann, and C. F. Ibáñez, ‘Growth differentiation factor 11 signals through the transforming growth factor-beta receptor ALK5 to regionalize the anterior-posterior axis.’, EMBO Rep., vol. 7, no. 8, pp. 831–7, 2006, doi: 10.1038/sj.embor.7400752.
[4] A. D. Jurberg, R. Aires, I. Varela-Lasheras, A. Nóvoa, and M. Mallo, ‘Switching axial progenitors from producing trunk to tail tissues in vertebrate embryos’, Dev. Cell, vol. 25, no. 5, pp. 451–462, 2013, doi: 10.1016/j.devcel.2013.05.009.
[5] A. Lozovska et al., ‘Tgfbr1 regulates lateral plate mesoderm and endoderm reorganization during the trunk to tail transition’. Mar. 19, 2024. doi: 10.7554/eLife.94290.1.
[6] J. Larsson et al., ‘Abnormal angiogenesis but intact hematopoietic potential in TGF-β type I receptor-deficient mice’, EMBO J., vol. 20, no. 7, pp. 1663–1673, 2001, doi: 10.1093/emboj/20.7.1663.
[7] C. Lin et al., ‘Delineating a Conserved Genetic Cassette Promoting Outgrowth of Body Appendages’, PLoS Genet., vol. 9, no. 1, pp. 1–12, 2013, doi: 10.1371/journal.pgen.1003231.
[8] M. J. Cohn, ‘Development of the external genitalia: Conserved and divergent mechanisms of appendage patterning’, Dev. Dyn., vol. 240, no. 5, pp. 1108–1115, May 2011, doi: 10.1002/dvdy.22631.
[9] J. D. Buenrostro, B. Wu, H. Y. Chang, and W. Greenleaf, ‘ATAC-seq: A Method for Assaying Chromatin Accessibility Genome-Wide’, Curr Protoc Mol Biol, vol. 48, no. 6, pp. 1197–1222, 2016, 10.1002/0471142727.mb2129s109
[10] J. Malkmus et al., ‘Spatial regulation by multiple Gremlin1 enhancers provides digit development with cis-regulatory robustness and evolutionary plasticity’, Nat. Commun., vol. 12, no. 1, p. 5557, Dec. 2021, doi: 10.1038/s41467-021-25810-1.
[11] R. D. Riddle, R. L. Johnson, E. Laufer, and C. Tabin, ‘Sonic hedgehog mediates the polarizing activity of the ZPA’, Cell, vol. 75, no. 7, pp. 1401–1416, Dec. 1993, doi: 10.1016/0092-8674(93)90626-2.
[12] A. Zúñiga et al., ‘Mouse limb deformity mutations disrupt a global control region within the large regulatory landscape required for Gremlin expression’, Genes Dev., vol. 18, no. 13, pp. 1553–1564, Jul. 2004, doi: 10.1101/gad.299904.
[13] C. L. Perriton, N. Powles, C. Chiang, M. K. Maconochie, and M. J. Cohn, ‘Sonic hedgehog Signaling from the Urethral Epithelium Controls External Genital Development’, Dev. Biol., vol. 247, no. 1, pp. 26–46, Jul. 2002, doi: 10.1006/dbio.2002.0668.