3rd Crick Beddington Symposium: Shared Roots and Divergent Paths in the Chick Spinal Cord
Posted by Alex Neaverson, on 26 March 2025
During the coffee break on the second day of the 3rd Crick Beddington Symposium, I approached Dr Giulia Boezio, a postdoc in the Briscoe lab at the Francis Crick Institute. As a fellow chick embryologist, I was already aware of her work, but I was intrigued to learn more about the genomic barcoding technique that she has adapted for use in our favourite model organism!
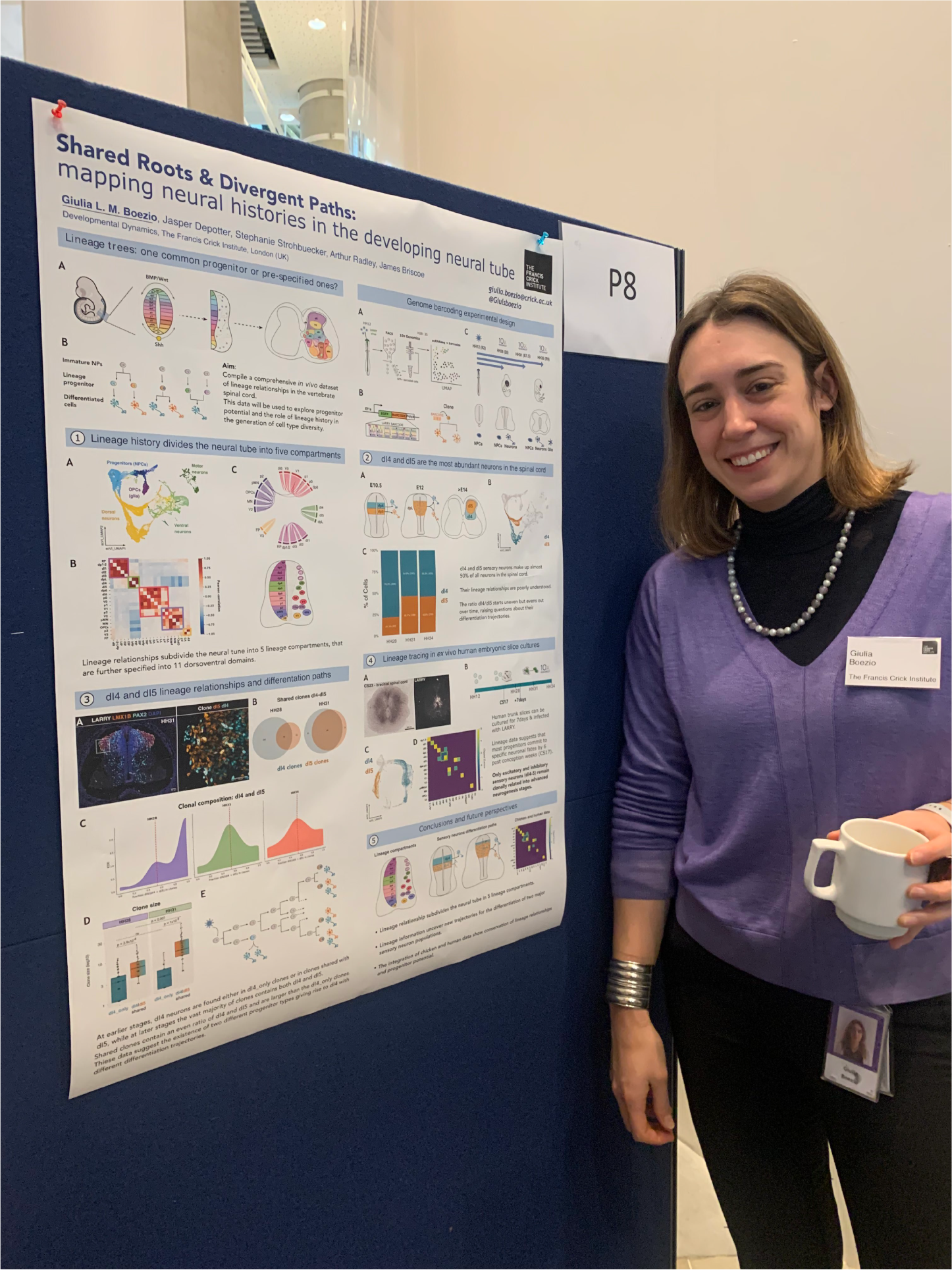
The Briscoe lab are interested in how the huge diversity of neuronal subtypes arise in the spinal cord. Different cell types originate from different positions along the dorsoventral axis, constituting 11 discrete domains of progenitor cells (Holguera and Desplan, 2018; Le Dréau and Martí, 2012; Sagner and Briscoe, 2019). But we still don’t know how these cell types arise – does one type of mature neuron come from one specific progenitor, or might two different mature neurons share a bi-fated progenitor? Or perhaps the differentiation paths converge – two different progenitors might ultimately give rise to the same final cell type. Giulia aims to shed light on these questions, which surprisingly had not been revisited in over 30 years (Leber et al., 1990; Leber and Sanes, 1991).
Giulia has adapted a genomic barcoding technique called LARRY (Lineage and RNA Recovery; Weinreb et al., 2020) – originally developed for cells in culture – for use in the chick embryo. Viral infection is used to deliver barcodes into the chick spinal cord at stage 12 (day 2 of development). Each barcode is unique, and is expressed alongside GFP, allowing the infected cells to be tracked over time. The embryos are incubated to develop for several days, before the mature cells are collected at one of three time points (stage 28, 31 and 35). They are then FACS sorted to obtain the GFP-expressing cells, and profiled using single cell RNAseq. After identifying which cluster corresponds to which cell type, Giulia can then see which barcodes are present in each cluster. Since every cell with the same barcode is derived from a single progenitor, this allows a retrospective lineage history to be assembled. I wondered how they can guarantee that each barcode is completely unique, but Giulia explained, “we have almost half a million barcodes in our library. This wouldn’t normally be enough, if we were infecting many, many cells. But the bug that actually turned out to be a (good) feature is that the infection rate is not very high.” This means that the chance of infecting two independent cells with the same barcode is extremely slim. She also checked across five separate embryos, and found no barcode overlap – so it is safe to assume that within one embryo, each barcode is unique.
From the single cell RNAseq data, she found that spinal cord cells group into five lineage compartments – meaning that progenitors will mostly contribute to cells in one of these five lineage compartments, and rarely contribute to more than one. Some cell types were more abundant than others – this is particularly true for the dorsal dI4 and dI5 neurons, which make up more than half of all the neurons in the entire spinal cord. These sensory neurons are involved in touch and pain sensation (Lai et al., 2016), and despite their abundance, are not very well studied. Giulia observed that their ratios changed over time; at stage 28, she saw many more dI4 than dI5 neurons, but by stage 31 this had equalised to roughly 50:50 – this makes perfect sense, since one is an excitatory neuron, and the other is an inhibitory neuron in the same circuit.
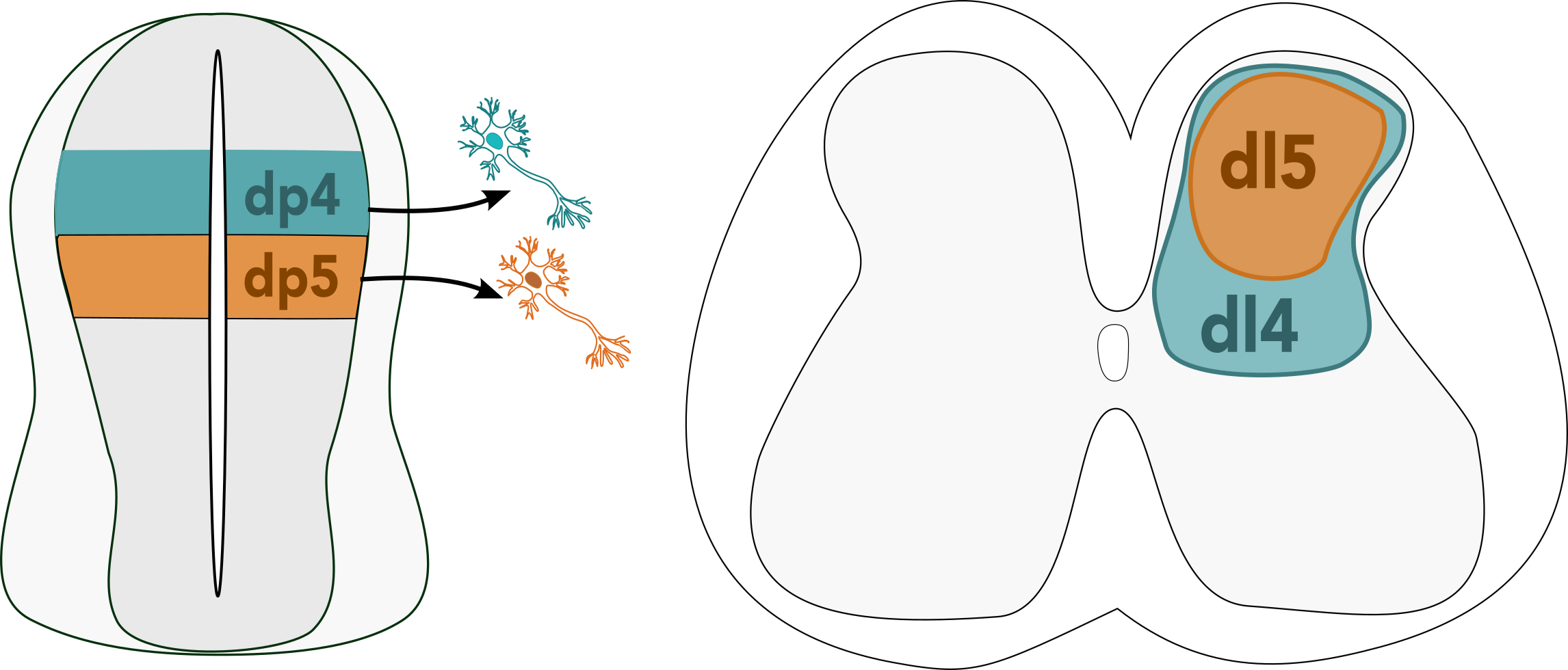
But how does this imbalance arise, and how does it correct itself later on? “Initially, when I saw this, I thought that the easiest explanation would be that the blue (dI4) cells are born earlier, and then eventually the orange (dI5) cells would catch up somehow. But we had to dig a bit deeper into the clonal data, and this explanation didn’t seem to fit”, Giulia said, after observing an initial peak of dI4 neurons, followed by a peak in both dI4 and dI5 neurons later in development. It turns out that at earlier stages, most dI4 neurons derive from clones that only produce dI4 neurons, while at later stages, the vast majority of clones produce an even ratio of dI4 and dI5 neurons. This suggests that there are two different progenitor types – one that only produces dI4 neurons, and one that produces both dI4 and dI5 in equal quantities. Giulia is currently working on fitting this data to different mathematical models to find a differentiation trajectory that might explain this result.
Does this system apply to other species? To find out, Giulia used ex vivo cultures of human embryonic trunk slices – a technique (which truly sounds like science fiction to me!) in which transverse sections of the spinal cord are grown using an air-liquid interface, exposed to cell culture media from the bottom, and the air from above. Work with human embryos is notoriously difficult, as you cannot control the availability, nor what stage will be available to work on. Giulia managed to obtain some 6 week old (CS17) human embryos – older than she would have liked, but she decided to go ahead with the experiment anyway. She infected the CS17 trunk slices with LARRY barcodes and cultured them for 7 days, collecting and profiling the cells in the same way as for the chick experiment. She found that dI4 and dI5 dominated over other neurons in terms of abundance, as she saw in the chick. There were very few shared clones between ventral neural cell types; this fits with the fact that neurogenesis occurs earlier in this compartment. Some clones were shared in the dorsal neural tube, but only between other neurons of the same type. The only exception was the dI4 and dI5 neurons, which still shared the vast majority of barcodes, meaning that at the time of infection, the decision to become dI4 or dI5 had not yet been made. This appears to be the latest lineage decision of any neuron in the spinal cord. Why might this be important? Giulia speculates that their proliferation as progenitors might help to ensure that the final ratio of dI4:dI5 is balanced, since there are diseases that can arise from an imbalance between these two neurons.
Giulia’s hypothesis is that there are two different groups of progenitors in the dorsal spinal cord, one that gives rise to dI4 neurons and one that maintains progenitors with bi-fated potential. The temporal sequence is still unclear; Giulia speculates that the different groups of progenitors may mature into neurons at different rates, or begin maturing at different times, eventually resulting in an approximate 1:1 ratio. At the moment, there is no data to show what happens between the dI4-dominant stage 28 and the restoration of balance at stage 31, so it is not possible to prove when or how this change happens. Giulia is currently working on fitting a model to her data in order to test this hypothesis as well as complementing her sequencing data with live imaging to fill the temporal gaps.
Stay tuned for more poster interviews coming soon!
References:
Holguera, I., Desplan, C., 2018. Neuronal specification in space and time. Science 362, 176–180. https://doi.org/10.1126/science.aas9435
Lai, H.C., Seal, R.P., Johnson, J.E., 2016. Making sense out of spinal cord somatosensory development. Development 143, 3434–3448. https://doi.org/10.1242/dev.139592
Le Dréau, G., Martí, E., 2012. Dorsal-ventral patterning of the neural tube: a tale of three signals. Dev Neurobiol 72, 1471–1481. https://doi.org/10.1002/dneu.22015
Leber, S., Breedlove, S., Sanes, J., 1990. Lineage, arrangement, and death of clonally related motoneurons in chick spinal cord. J Neurosci 10, 2451–2462. https://doi.org/10.1523/JNEUROSCI.10-07-02451.1990
Leber, S.M., Sanes, J.R., 1991. Lineage analysis with a recombinant retrovirus: application to chick spinal motor neurons. Adv Neurol 56, 27–36.
Sagner, A., Briscoe, J., 2019. Establishing neuronal diversity in the spinal cord: a time and a place. Development 146, dev182154. https://doi.org/10.1242/dev.182154
Weinreb, C., Rodriguez-Fraticelli, A., Camargo, F.D., Klein, A.M., 2020. Lineage tracing on transcriptional landscapes links state to fate during differentiation. Science 367, eaaw3381. https://doi.org/10.1126/science.aaw3381