New insights into sex differences in Drosophila development and physiology
Posted by Elizabeth Rideout, on 27 May 2016
Bruno Hudry and Elizabeth J. Rideout
Male and female fruit flies differ in many aspects of development and physiology. For example, males and females differ in abdominal pigmentation, sex comb formation, courtship behaviours, the development and wiring of the central nervous system, internal reproductive organs, and genital morphology. Many elegant studies on the genes that regulate sex differences in these traits have led to the prevailing model of sex determination in Drosophila where the number of sex chromosomes determines male or female sexual identity1,2.
Briefly, the presence of two X chromosomes in female cells causes the production of an X-derived protein called Sex-lethal3. Sxl is a splicing factor, and introduces a splice into the pre-mRNA of an autosomal gene called transformer (tra) that allows a functional protein to be produced4-6. Like Sxl, Tra is a splicing factor, and together with its co-factor transformer2 (tra2), binds to the pre-mRNA of two genes: doublesex (dsx) and fruitless (fru)7-10. Tra-dependent splicing of dsx pre-mRNA causes the production of an mRNA encoding the female-specific isoform of Dsx protein (DsxF). In contrast, Tra binding to fru pre-mRNA introduces a stop codon into transcripts derived from the P1 promoter, and no Fru P1 proteins are produced in females. In males, with one X chromosome, Sxl is not induced, and consequently no functional Tra protein is produced. Lack of Tra means that dsx and fru P1 pre-mRNA undergo default splicing, and the male-specific isoforms of each protein are made (DsxM and FruM).
This sex determination cascade explains most aspects of sexual development and reproduction. However, male and female flies also differ in body size, stress responses, immunity, physiology, and lifespan11-14. Yet the mechanisms underlying sex differences in these additional traits remain unclear. The focus of this blog post is to highlight the findings from our two recent papers on sex differences in Drosophila body size (Rideout et al. 201515) and adult intestinal stem cell homeostasis (Hudry et al. 201616). We will review how these findings advance our understanding of the genetic control of sexual identity in flies, and the use of Drosophila as a model to study the molecular mechanisms underlying sexual dimorphism in body size and gut physiology.
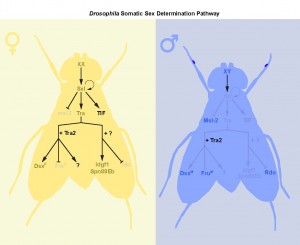
Sex determination: different tissues, different rules
Studies on courtship behaviour, abdominal pigmentation, and sex comb formation show a clear role for the canonical Sxl→tra→dsx/fru splicing cascade in the creation of sex differences3,17. However, our findings, together with data from other studies18,
demonstrates that the genetic control of sexual identity involves additional downstream effector pathways (Fig. 1). These pathways operate in different tissues, and at different stages of the life cycle, suggesting that the process of sex determination in flies is more complex, and includes more genes, than the central core of Sxl/tra/dsx/fru (Fig. 2).
Beyond dsx and fru: new downstream targets of tra
Although there is growing evidence to support a tra-independent branch of the sex determination pathway downstream of Sxl, whether an additional branch exists downstream of tra is unclear. Previous studies on dissatisfaction (dsf), a gene with effects on sex determination in males and females, suggested a tra-dependent, dsx-independent branch of the sex determination pathway19. Yet the authors were not able to rule out the possibility that dsf may be target of fru20. Further, there was no evidence supporting sex differences in dsf expression, transcript levels, or splicing. Our two papers now provide concrete evidence of the existence of a tra-dependent, but dsx-/fru-independent, branch of the sex determination pathway in two different contexts: larval body growth, and adult intestinal stem cell (ISC) homeostasis.
In Rideout et al. (2015) we show that tra promotes the increased body size of female flies. Loss of Tra in female larvae decreases body size, whereas ectopic expression of Tra in male larvae increases body size. Specifically, we found that Tra functions in the larval fat body to control overall body size in a non-cell-autonomous manner, and that these effects on body size were reproduced in larvae with fat-specific loss of Tra2. Surprisingly, body size was unaffected in dsx mutants, females ectopically expressing FruM, and in males lacking FruM. Thus tra/tra2, acting independently of fru and dsx, control male-female differences in body size.
Similarly, in Hudry et al. (2016), we show that tra promotes higher ISC proliferation rate in females. This sex difference in adult stem cell divisions controls sex differences in organ size, plasticity during reproduction, and response to tumorigenic insult. Using a series of rescue, and gain- and loss-of-function experiments with dsxF transgenes, dsx/fru mutants, and RNAi transgenes, we ruled out both direct and indirect actions of dsx and fru on ISC proliferation.
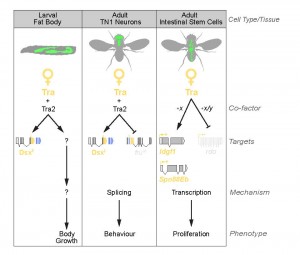
Together, our studies provide strong evidence that Tra’s effects on body size and ISC proliferation are independent of both dsx and fru (Fig. 2). Interestingly, though our findings converge on the existence of a fru-/dsx-independent branch of the sex determination pathway downstream of tra, we found important differences in the molecular mechanisms of tra’s effects on larval growth and ISC proliferation. For example, in larvae, the dsx-/fru-independent effects of tra on body size require tra’s partner tra2. In contrast, tra2 is not required for tra’s effects on ISC proliferation in the adult gut. How tra activity acquires tissue- and cell-type-specificity is not yet understood and emerges as an important new question.
One possibility is that tra acts via co-operation with tissue-specific partners. For example, one study found that among 217 Drosophila genes with Entrez Gene identifiers related to RNA-binding proteins (including splicing regulators), 83 produce multiple transcripts21. The temporal and spatial regulation of the production of these different isoforms is far from being elucidated. However, among these 217 RNA-binding proteins, 35 display very specific expression pattern in adult tissues. Some of these alternative splice variants, or tissue-specific RNA binding proteins, could be context-specific regulators of tra-mediated splicing.
Alternatively, tra might influence ISC proliferation and body size via regulation of other aspects of RNA metabolism. Tra is a member of the SR family of proteins, which play diverse roles in RNA metabolism, including effects on transcript stability, mRNA translation, and transcriptional regulation of gene expression22. A non-splicing function for Tra in ISCs is supported by lack of Tra/Tra2 binding sites near tra-regulated splice junctions in adult ISCs. On the other hand, a genome-wide search successfully identified 18 candidate targets with multiple Tra/Tra2 sites, including tra’s known targets dsx, fru, and Sxl. Indeed, the three functional targets of tra identified in adult ISCs are regulated at the level of mRNA expression rather than splicing, suggesting one explanation for why tra2 is not required for adult ISC proliferation.
Drosophila as a model for sex differences in development and physiology
Sex differences in body size, stress responses and physiology are common to many animals other than flies, including mammals. Decades of research have examined the important contribution that sex hormones make to sex differences in traits such as adiposity and stem cell biology. For example, estrogen in female mammals causes a smaller body size, limits visceral fat accumulation, and increases haematopoietic stem cell self-renewal in females in both homeostatic conditions and during pregnancy23-25.
However, when sex hormones are absent, data from new mouse models shows that chromosomal sex (e.g. XX vs XY) dictates male-female differences in body size and adiposity. For example, in the Four Core Genotypes mouse model (FCG), which allows the effects of sex chromosomes to be directly assessed independently of sex hormones, gonadectomized XX animals achieve a larger body weight than XY animals26. This mirrors the situation in flies, where XX females are larger than XY males. Thus, in the absence of sex hormones, the trends in body size between mammals and flies are remarkably similar.
At present, it remains unclear how sex chromosomes affect body size and adiposity in mice. Flies may therefore provide important insight into the molecular mechanisms underlying these sex hormone-independent effects on body size and adiposity. For example, an important finding from Rideout et al. (2015) is that females achieve a larger body size at least in part due to increased secretion of Drosophila insulin-like peptide 2. Intriguingly, XX FCG mice have higher fasting levels of insulin26. Since the insulin pathway is an important regulator of body weight and adiposity, similar pathways may be involved in mediating the effects of sex on these traits in both flies and mice.
Over the past two decades, Drosophila has provided vital information on the molecular and physiological mechanisms responsible for determining growth during development and final body size31. Drosophila ISCs have more recently emerged as a good model to investigate adult somatic stem cell functions and regulation in vivo32,33. Recent studies using the fly model have identified many conserved intrinsic factors and extrinsic niche signals that drive the proliferation, self-renewal, and differentiation of ISCs34. In addition, a recent study demonstrated that the sex of the gut mediates the sex-biased responses of males and females to lifespan extension by dietary restriction35. We therefore suggest that flies present an excellent model to dissect the molecular mechanisms underlying sex differences in diverse aspects of development and physiology.
References
- Bridges, C.B. Triploid Intersexes in Drosophila Melanogaster. Science 54, 252-4 (1921).
- Erickson, J.W. & Quintero, J.J. Indirect effects of ploidy suggest X chromosome dose, not the X:A ratio, signals sex in Drosophila. PLoS Biol 5, e332 (2007).
- Cline, T.W. & Meyer, B.J. Vive la difference: males vs females in flies vs worms. Annu Rev Genet 30, 637-702 (1996).
- Butler, B., Pirrotta, V., Irminger-Finger, I. & Nothiger, R. The sex-determining gene tra of Drosophila: molecular cloning and transformation studies. EMBO J 5, 3607-13 (1986).
- Boggs, R.T., Gregor, P., Idriss, S., Belote, J.M. & McKeown, M. Regulation of sexual differentiation in D. melanogaster via alternative splicing of RNA from the transformer gene. Cell 50, 739-47 (1987).
- Inoue, K., Hoshijima, K., Sakamoto, H. & Shimura, Y. Binding of the Drosophila sex-lethal gene product to the alternative splice site of transformer primary transcript. Nature 344, 461-3 (1990).
- Ryner, L.C. et al. Control of male sexual behavior and sexual orientation in Drosophila by the fruitless gene. Cell 87, 1079-89 (1996).
- Heinrichs, V., Ryner, L.C. & Baker, B.S. Regulation of sex-specific selection of fruitless 5′ splice sites by transformer and transformer-2. Mol Cell Biol 18, 450-8 (1998).
- Inoue, K., Hoshijima, K., Higuchi, I., Sakamoto, H. & Shimura, Y. Binding of the Drosophila transformer and transformer-2 proteins to the regulatory elements of doublesex primary transcript for sex-specific RNA processing. Proc Natl Acad Sci U S A 89, 8092-6 (1992).
- Hoshijima, K., Inoue, K., Higuchi, I., Sakamoto, H. & Shimura, Y. Control of doublesex alternative splicing by transformer and transformer-2 in Drosophila. Science 252, 833-6 (1991).
- Alpatov, W.W. Phenotypical variation in body and cell size of Drosophila melanogaster. Biological Bulletin 58, 85-103 (1930).
- Taylor, K. & Kimbrell, D.A. Host immune response and differential survival of the sexes in Drosophila. Fly (Austin) 1, 197-204 (2007).
- Neckameyer, W.S. & Matsuo, H. Distinct neural circuits reflect sex, sexual maturity, and reproductive status in response to stress in Drosophila melanogaster. Neuroscience 156, 841-56 (2008).
- Magwere, T., Chapman, T. & Partridge, L. Sex differences in the effect of dietary restriction on life span and mortality rates in female and male Drosophila melanogaster. J Gerontol A Biol Sci Med Sci 59, 3-9 (2004).
- Rideout, E.J., Narsaiya, M.S. & Grewal, S.S. The Sex Determination Gene transformer Regulates Male-Female Differences in Drosophila Body Size. PLoS Genet 11, e1005683 (2015).
- Hudry, B., Khadayate, S. & Miguel-Aliaga, I. The sexual identity of adult intestinal stem cells controls organ size and plasticity. Nature 530, 344-8 (2016).
- Billeter, J.C., Rideout, E.J., Dornan, A.J. & Goodwin, S.F. Control of male sexual behavior in Drosophila by the sex determination pathway. Curr Biol 16, R766-76 (2006).
- Evans, D.S. & Cline, T.W. Drosophila switch gene Sex-lethal can bypass its switch-gene target transformer to regulate aspects of female behavior. Proc Natl Acad Sci U S A 110, E4474-81 (2013).
- Finley, K.D. et al. Dissatisfaction encodes a tailless-like nuclear receptor expressed in a subset of CNS neurons controlling Drosophila sexual behavior. Neuron 21, 1363-74 (1998).
- Shirangi, T.R. & McKeown, M. Sex in flies: what ‘body–mind’ dichotomy? Dev Biol 306, 10-9 (2007).
- Hartmann, B. et al. Distinct regulatory programs establish widespread sex-specific alternative splicing in Drosophila melanogaster. RNA 17, 453-68 (2011).
- Shepard, P.J. & Hertel, K.J. The SR protein family. Genome Biol 10, 242 (2009).
- Gambacciani, M. et al. Body weight, body fat distribution, and hormonal replacement therapy in early postmenopausal women. J Clin Endocrinol Metab 82, 414-7 (1997).
- Grove, K.L., Fried, S.K., Greenberg, A.S., Xiao, X.Q. & Clegg, D.J. A microarray analysis of sexual dimorphism of adipose tissues in high-fat-diet-induced obese mice. Int J Obes (Lond) 34, 989-1000 (2010).
- Nakada, D. et al. Oestrogen increases haematopoietic stem-cell self-renewal in females and during pregnancy. Nature 505, 555-8 (2014).
- Chen, X. et al. The number of x chromosomes causes sex differences in adiposity in mice. PLoS Genet 8, e1002709 (2012).
- Markle, J.G. et al. Sex differences in the gut microbiome drive hormone-dependent regulation of autoimmunity. Science 339, 1084-8 (2013).
- Steegenga, W.T. et al. Sexually dimorphic characteristics of the small intestine and colon of prepubescent C57BL/6 mice. Biol Sex Differ 5, 11 (2014).
- Cook, M.B. et al. Sex disparities in cancer incidence by period and age. Cancer Epidemiol Biomarkers Prev 18, 1174-82 (2009).
- El Ouaamari, A. et al. SerpinB1 Promotes Pancreatic beta Cell Proliferation. Cell Metab 23, 194-205 (2016).
- Edgar, B.A. How flies get their size: genetics meets physiology. Nat Rev Genet 7, 907-16 (2006).
- Micchelli, C.A. & Perrimon, N. Evidence that stem cells reside in the adult Drosophila midgut epithelium. Nature 439, 475-9 (2006).
- Ohlstein, B. & Spradling, A. The adult Drosophila posterior midgut is maintained by pluripotent stem cells. Nature 439, 470-4 (2006).
- Li, H. & Jasper, H. Gastrointestinal stem cells in health and disease: from flies to humans. Dis Model Mech 9, 487-99 (2016).
- Regan, J.C. et al. Sex difference in pathology of the ageing gut mediates the greater response of female lifespan to dietary restriction. Elife 5, (2016).