Shaping the embryo towards gastrulation
Posted by Christos Kyprianou, on 13 May 2020
Kyprianou, C., Christodoulou, N., Hamilton, R.S. et al. Basement membrane remodelling regulates mouse embryogenesis. Nature (2020). doi.org/10.1038/s41586-020-2264-2
Morphogenesis is a complicated network of processes that involve cell shape changes, cell movements and division patterns. While these are part of the intricate game that gives rise to tissue shapes, there is another element that is now emerging as a major player. A supra-cellular physical scaffold that directs sculpting at the tissue level; the basement membrane (BM).
To put this statement to the test, in our recent paper [1] we have investigated how the developing mouse embryo takes shape through a ‘primitive’ and newly forming basement membrane that surrounds the embryo, at a time when it’s exponentially growing.
Once the mouse embryo implants, it transforms into what we call an ‘egg cylinder’ which refers to its elongated cylindrical shape (Figure 1).
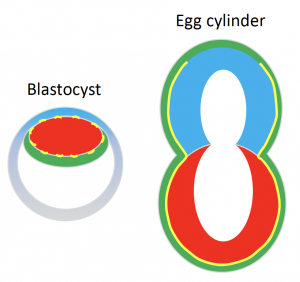
The elongated shape of the egg cylinder is maintained while it grows in size. The very fact that shape remains virtually unaltered during growth baffled us however. We suspected that there must be a mechanism in place that actively maintains the shape somehow. Our suspicions started to lean towards the BM when its complete dissolution with Collagenase IV affected the embryo shape.
We know quite a bit about the signalling niche the BM creates. It’s a laminin- and collagen-rich extracellular matrix that surrounds the embryo and induces polarisation of the cells it’s in contact with through integrin-mediated signalling. In turn this drives processes like cavity formation and extension that gives egg cylinder its characteristic hollowed appearance (Figure 1)[2]. But how about its physical properties and its potential role in physically guiding (and maintaining) shape?
The ‘net-like’ basement membrane
Inspired by the work of Kenneth Yamada and Donald Ingber on the architecture of BM we went on to investigate its appearance [3,4]. What we discovered was a striking difference in how BM is laid down in the two halves of the embryo along the proximo-distal axis (long axis). Specifically, the embryonic half (EPI) is heavily perforated in contrast to the extraembryonic half (ExE) (Figure 2). This architecture has been observed before in growing limb buds and was suggested that this allows directional bud growth and subsequent branching morphogenesis. Similarly, in this study we argued that this perforated morphology of the BM in the embryonic portion of the embryo allows embryo growth in a directional manner i.e. growth towards the side of least resistance (in this case the distal portion of the embryo) while maintaining at the same time the overall BM integrity and the embryo’s elongated shape.
Is it possible that this ‘net-like’ morphology of the BM is a way of decreasing physical constraints at the side of ‘desired’ growth direction? To answer this, we would need to ‘homogenise’ the BM by ‘filling up’ these perforations and re-examining embryo growth.
But first we would need to abrogate whatever mechanism creates these perforations in the first place.
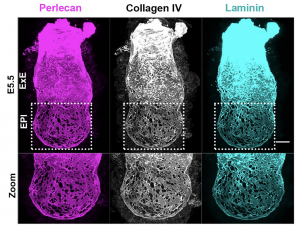
Finding an AVEnue to elucidate the mechanism
The natural process itself gave us our most valuable hint towards understanding the underlying mechanism of perforation generation. The natural process I am referring to is the establishment of the anterior-posterior axis, which takes place during these early stages of development. A specialised population of cells in the outer layer of the embryo (the visceral endoderm; VE) emerge at the distal tip and start migrating to one side to establish the anterior portion. These cells are called (you guessed it) anterior visceral endoderm (AVE). To our surprise, the BM underlying these cells loses its perforated morphology and by the time AVE has populated the future anterior side of the embryo, the perforation distribution is heavily skewed towards the posterior. Abrogation of AVE movement in a RhoA VE-specific knockout also affects the asymmetric redistribution of BM perforations, in line with the suspected impact of AVE on the underlying BM (Figure 3). This was our breakthrough in not only understanding the mechanism of forming the perforations but also their role in the pre-gastrula stages. But first let us consider the mechanism and how this observation led us to solving it.
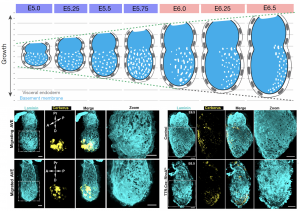
The AVE brings about its anteriorising and posteriorising effect by modulating Nodal activity. It secretes Nodal inhibitors (namely Cerberus and Lefty) in its vicinity confining Nodal activity to the future posterior. In turn, Nodal drives mesendodermal specification in the posterior and kicks off later development with the formation of the three germ layers. In the context of our question, however, it suggested that Nodal inhibition may be behind the loss of BM perforations under AVE. Both pharmacological inhibition and genetic knockout of Nodal presented the same phenotype; a solid unperforated BM.
Nodal is not-all there is to it: MMPs are the MVPs
Next question was to identify the downstream effectors of this process. Naturally, we suspected matrix metalloproteinases (MMPs) might be playing a role in this, due to their collagenolytic properties and dissolving extracellular matrix to facilitate processes in cancer pathogenesis like metastasis. Knowing that BM perforation was a Nodal-dependent mechanism we established MMP candidate eligibility criteria based on: 1) temporal expression (during stages of development we were investigating i.e. early post-implantation to pre-gastrula stages), 2) spatial expression (embryonic portion of the embryo and posterior expression at pre-gastrula stages) and 3) response to Nodal.
Thanks to the incredibly useful single cell sequencing and ChIP-seq databases available from previous studies we were able to mine them for the MMP candidates meeting these criteria [5,6]. This gave us two candidates, MMP2 and MMP14.
To upgrade their “candidate” status to the “player” status, we confirmed that they fit all three criteria by RNAscope (FISH), directly looking at their distribution and response to Nodal inhibition. To functionally confirm them, we inhibited these MMPs using a cocktail of MMP inhibitors and phenocopied Nodal inhibition and perturbation. BM lost its perforated morphology and the embryo growth was significantly reduced in response to it.
To condense all this in one sentence: “The growth of the embryo is regulated by modulating the physical constraints, imposed by the surrounding BM, through perforations, in a Nodal-dependent MMP-mediated fashion”.
But what’s the connection of this process with gastrulation?
Revisiting the ‘Run in the stocking’ model
Gastrulation is a super-process, if you will, that involves a number of processes taking place simultaneously and co-ordinately, to achieve the formation of the three germ layers and the extraembryonic mesoderm for the extraembryonic membranes. While most of us would recognise the onset of gastrulation at the time when we observe the first mesendoderm cells forming at the posterior of the embryo, it’s merely the preparation for gastrulation.
What kicks things off, is the breaking of the BM under the mesendoderm cells and initiation of epithelial-to-mesenchymal transition with subsequent ingression in the layers below. So, we can argue that the cells in the posterior epiblast are specified into mesendoderm in preparation for their journey to the lower layers to establish the germ layers. Similarly, we suggest that the BM may go through a similar preparation phase becoming prone to breakage and ultimately be breached at the right time when mesendoderm cells have been specified and ready to ingress.
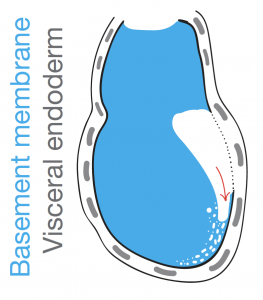
The suggestion of a BM pre-patterning for gastrulation came with the observation of perforation redistribution to the future posterior upon establishment of the anterior-posterior axis. As described earlier, the anterior signalling centre, AVE, through local inhibition of Nodal activity, creates a posterior “perforation domain” in the BM. This domain is established even before mesendoderm specification and constitutes now a first and novel posteriorising characteristic. We hypothesised that an asymmetrically perforated BM will preferentially be breached on the side of least resistance and thus the posterior BM. By investigating the successive steps of gastrulation and primitive streak extension with progressive breaching of BM we saw that the perforations persist even during the process of gastrulation, “paving the way”, in a sense, for a controlled and ordered ingression of mesendoderm cells.
What we saw was consistent with a “run in the stocking” model, as first proposed by Donald Ingber [4] in which a “weakened” BM domain will expand in response to forces imposed by cell proliferation and tissue growth. In our case this weakened BM is the pre-patterned posterior BM which, much like a tear in a stocking, will progressively break as cells force their way through the breach (Figure 4). To confirm the importance of the perforation domain along the prospective primitive streak, we inhibited MMPs prior to gastrulation to homogenise the BM. We noticed mesendoderm cells being specified but failing to ingress to the extent of our controls, trapping them in the epiblast and in some cases seeing them being extruded and dying in the pro-amniotic cavity.
Final thoughts
The discovery of BM perforations in the early post-implantation mouse embryo has given a new, more physical, perspective of early morphogenesis. The groups of David Bilder, Barry Thompson, Magali Suzanne and Kenneth Yamada are some of the leading teams looking at BM-directed tissue morphogenesis and have published exciting research that stretches from fruit flies to mice [3,7,8,9,10]. While the mode of basement membrane remodelling in different species and tissues can be different (perforations, collagen fibril arrangement or complete dissolution) it seems that the principle of this morphogenetic mechanism is evolutionary conserved. Further study promises more insight in how complicated structures and shapes have evolved. This is an area where computational modelling of BM dynamics in shape-forming can supplement the cellular dynamics field of study in morphogenesis.
The current work in mouse has translational value as well. A similar mechanism could be in operation in human embryos during implantation, a stage when embryos rapidly expand and transform but also a stage when many human pregnancies fail.
I am looking forward to see how this area develops and discuss with anyone interested.
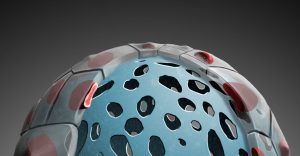
[1] Kyprianou, C., Christodoulou, N., Hamilton, R. S., Nahaboo, W., Boomgaard, D. S., Amadei, G., & Migeotte, I. (2020). Basement membrane remodelling regulates mouse embryogenesis. Nature, (March 2019). https://doi.org/10.1038/s41586-020-2264-2
[2] Christodoulou, N., Kyprianou, C., Weberling, A., Wang, R., Cui, G., Peng, G., … and Zernicka-Goetz, M. (2018). Sequential formation and resolution of multiple rosettes drives embryo remodeling after implantation. Nature Cell Biology. https://doi.org/10.1038/s41556-018-0211-3
[3] Harunaga, J. S., Doyle, A. D., & Yamada, K. M. (2014). Local and global dynamics of the basement membrane during branching morphogenesis require protease activity and actomyosin contractility. Developmental Biology, 394(2), 197–205. https://doi.org/10.1016/j.ydbio.2014.08.014
[4] Moore, K. A., Polte, T., Huang, S., Shi, B., Alsberg, E., Sunday, M. E., & Ingber, D. E. (2005). Control of basement membrane remodeling and epithelial branching morphogenesis in embryonic lung by Rho and cytoskeletal tension. Developmental Dynamics, 232(2), 268–281. https://doi.org/10.1002/dvdy.20237
[5] Cheng, S., Pei, Y., He, L., Peng, G., Reinius, B., Tam, P. P. L., … Deng, Q. (2019). Single-Cell RNA-Seq Reveals Cellular Heterogeneity of Pluripotency Transition and X Chromosome Dynamics during Early Mouse Development. Cell Reports, 26(10), 2593-2607.e3. https://doi.org/10.1016/j.celrep.2019.02.031
[6] Wang, Q., Zou, Y., Nowotschin, S., Kim, S. Y., Li, Q. V., Soh, C. L., … Massagué, J. (2017). The p53 Family Coordinates Wnt and Nodal Inputs in Mesendodermal Differentiation of Embryonic Stem Cells. Cell Stem Cell, 20(1), 70–86. https://doi.org/10.1016/j.stem.2016.10.002
[7] Haigo, S. L., & Bilder, D. (2011). Global Tissue Revolutions in a Morphogenetic Movement Controlling Elongation. Science, 331(6020), 1071–1074. https://doi.org/10.1126/science.1199424
[8] Crest, J., Diz-Muñoz, A., Chen, D. Y., Fletcher, D. A., & Bilder, D. (2017). Organ sculpting by patterned extracellular matrix stiffness. ELife, 6, 1–16. https://doi.org/10.7554/eLife.24958
[9] Del-Carmen Diaz-De-La-Loza, M., Loker, R., Mann, R. S., & Thompson, B. J. (2020). Control of tissue morphogenesis by the HOX gene Ultrabithorax. Development (Cambridge), 147(5), 1–13. https://doi.org/10.1242/dev.184564
[10] Proag, A., Monier, B., & Suzanne, M. (2019). Physical and functional cell-matrix uncoupling in a developing tissue under tension. Development (Cambridge), 146(11). https://doi.org/10.1242/dev.172577
Mich fasziniert seit Jahren die Totipotenz der pflanzlichen Zelle.
Wesshalb sind somatische pflanzliche Zellen totipotent
Und somatische tierische Zellen eben nicht.
Suppose I take two plates of iPSCs 2D on matrigel. I treat them both with growth factors normally secreted by the hypoblast. To one of the wells I meticulously perforate the matrigel coating with the tip of a P10. Will the perforated well develop into the mesoendoderm? and the non perforated into the ectoderm?