Salamander Models in Cross-Disciplinary Research – Meeting Report
Posted by ryankerney, on 18 September 2018
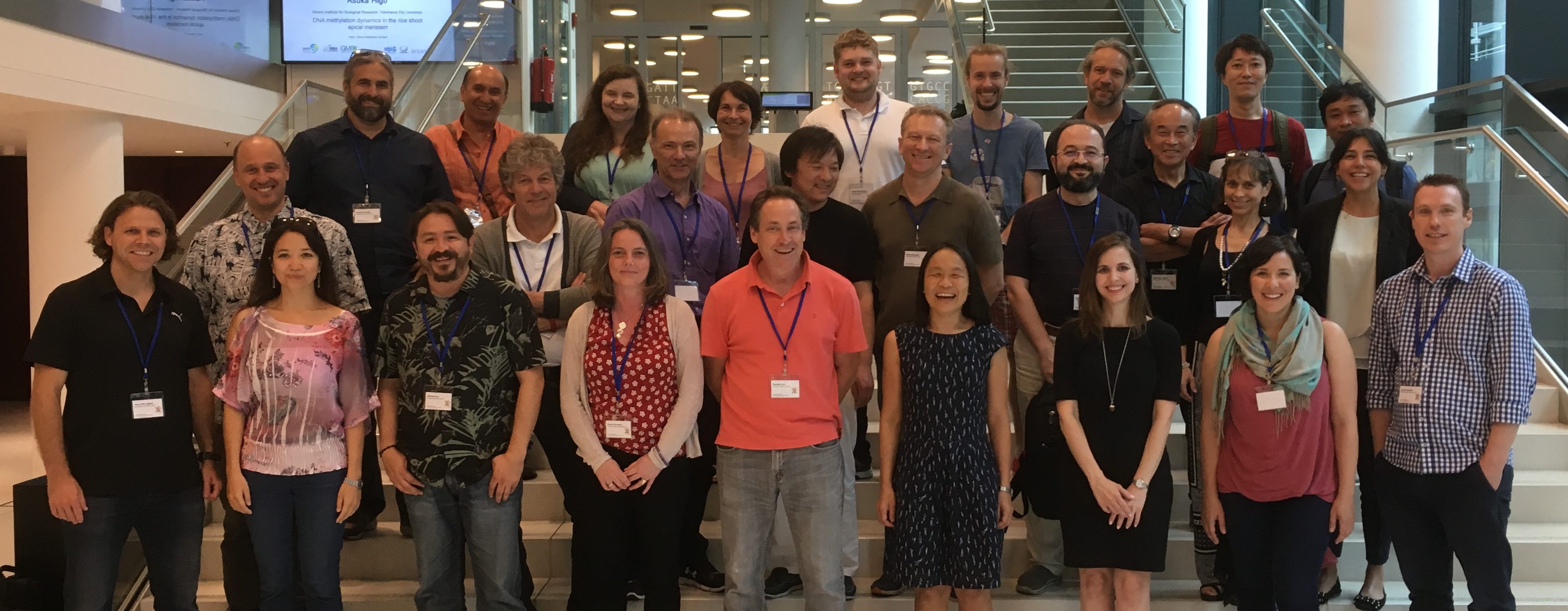
Vienna, Austria
July 2018
The use of salamanders in regeneration and developmental research has a long history filled with luminaries of the life sciences. Thomas Hunt Morgan, Hans Spemann and Hilde Mangold, Ross Granville Harrison, Inez Whipple Wilder, and August Weismann all employed salamanders in their work 1,2. Current experimental research on salamanders largely focuses on studies of regeneration. Their abilities to regenerate limbs, brain, kidney, heart, and tail3 is enviable from the perspective of our limited human regenerative abilities. There is a large and growing toolkit of experimental manipulations, mutant and transgenic lines, and genomic resources that have advanced the use of salamanders to probe critical questions on the evolution of regeneration and its loss. These offer opportunities to discover mechanisms that could lead to new therapies for tissue repair.
The first “Salamander Models in Cross-Disciplinary Biological Research” meeting, organized by Elly Tanaka, Jessica Whited, Karen Echeverri, and Randal Voss, was held this past July (2018) at the Research Institute of Molecular Pathology (IMP) in Vienna, Austria. The meeting was hosted by the intrepid Dr. Tanaka, who is a Senior Scientist at the IMP and a leader in the regenerative biology field. This was a gathering of principal investigators working on various aspects of salamander regeneration, development, genetics, and genomics. The major goals included reviewing recent advances in research tools, along with nuanced tips for employing them, while also establishing a master “to-do” list for the field. Another objective was to lay the groundwork for organizing future salamander meetings intended for the broader community, including postdoctoral and pre-doctoral trainees and potentially representatives from funding agencies.
The Salamanders
Unlike “the” worm or “the” fly, there are multiple salamander models used in molecular studies of development and regeneration. These prominently include the Mexican axolotl (Ambystoma mexicanum), the Iberian ribbed newt (Pleurodeles waltl), the Japanese newt (Cynops pyrrhogaster) and to a lesser extent the North American eastern newt (Notophthalmus viridescens) and several species of lungless salamanders (Plethodontidae). Currently the most commonly used model is the axolotl, which has the longest captive history of any laboratory animal1. This history includes the importation of a founder population to Paris in 1864, some of which contained the mutant “white” phenotype (an edn3 mutant), and deliberate introgression of an A. tigrinum locus found in 1962, which confers albinism through a tyrosinase mutation4,5. These salamander species are representatives of three families (Ambystomatidae, Plethodontidae, and Salamandridae – the newts) out of the ten extant salamander families, which likely had extensive limb regenerative abilities at the base of the their clade6.
Genomics
Our sessions started with a review of the impressive new work in salamander genomics. Recent published genomes for both the Iberian ribbed newt (Pleurodeles waltl7) and Mexican axolotl (Ambystoma mexicanum8) provide tremendous new resources for the field. The axolotl genome is roughly ten times the size of the human genome, making it the largest genome to be sequenced and assembled to date (sorry loblolly pine). The publication of both of these genomes promises to help resolve the loci of multiple established mutant lines5, and offers the opportunity to establish future forward and reverse genetic screens that will improve our mechanistic understanding of regenerative processes. The community identified additional work needed to make consistent annotations, resolving 5’ ends of genes, and approaching a chromosome scale resolution of contiguous sequence. The latter was recently made available through a bioRxiv preprint from the Smith and Voss labs at University of Kentucky. The lack of whole-genome sequences in salamanders has been a major impediment to the field, and though the assemblies will still require extensive refinements, having these resources should prove to be enormously beneficial to labs currently working with these species as well as those interested in starting salamander research.
Tansgenics and Genome Editing
Genome editing approaches are now available for the axolotl9,10, the Iberian newt11,7 and the Japanese fire-bellied newt Cynops pyrrhogaster12 using TALEN and CRISPR-based approaches. The most recent protocols developed by Ji-Feng Fei13 (now at the South China Normal University) for knock-out and knock-in approaches were reviewed. Current best practice techniques focused on improving knock-in strategies without relying on homology-directed repair. These include targeting introns for knock-in’s, screening injected embryo knockouts for efficient guide RNA’s prior to knock-ins, and non-homologous end joining approaches with “ORF Baits.”
The advent of CRISPR and TALEN approaches to genome editing in Pleurodeles was reviewed by Ken-Ichi Suzuki from Hiroshima University. The Suzuki lab is currently working to identify a ROSA-like locus for constitutive expression of knock-in constructs that would also provide a “safe harbor” for exogenous DNA. The intent of this approach is to develop a site that would both be minimally disruptive to normal cellular physiology while experiencing minimal interference from histone modifications in different cell lineages.
A wide range of transgenic and CRISPR edited lines are becoming available, especially in the axolotl. These include constitutive RFP and GFP reporter lines (available from the University of Kentucky’s Ambystoma Stock Center – AGSC), a pax7-mcherry muscle satellite cell marker, a neuronal marker, and a brainbow axolotl14. Discussions focused on prioritizing existing stocks and facilitating their dissemination through the AGSC (primarily in North America), Medipol University, Max Planck Dresden, and MPI (in Europe). The need to pursue financial resources to enable more extensive repository functions for salamanders, including cryopreservation to biobank lines, was also addressed. A long-term goal of the community is to secure resource funding for these types of valuable operations, which will be necessary to advance discoveries in regenerative biology through this growing research community.
Temporal control of transgene expression
Many genes implicated in regenerative processes have pleiotrophic roles in early development. These make knock out experiments in studying adult regeneration difficult as they can be embryonic lethal. Therefore both temporal and spatial control of gene expression is critical for furthering regeneration research.
There are several creative approaches available to address this potential stumbling block. These include inducible cre-lox systems15, constitutive cas-9 expression in genomic “safe harbors” with drug inducible guide RNA’s, and viral delivery of foreign transgenes into regenerative blastemas161718. The latter approach has been championed by both Jessica Whited’s group at Harvard University and the Tanaka lab at MPI. These pseudotyped retroviruses have tremendous potential for further labeling and functional studies of the limb blastema, without raising transgenic embryos or modifying the expression of pleiotrophic genes outside the limb.
Open discussions evaluating approaches and avenues for future research allowed individual labs to share their own research objectives with a collective eye toward developing critical methodologies to advance the community.
Resources needed for the field
The real value of this PI-focused meeting was to allow researchers to define limiting resources they found most pressing for the field. Several additional needs were identified in addition to continued improvements to genome annotations and the temporal control of gene expression. These included detailed histological atlases of regeneration, master lists of validated antibodies, and more stable cell lines for in vitro experiments. One of the most obvious resources needed was the continued communication between labs with subsequent salamander research conferences that should continue to strengthen this growing research community.
Coordination and prioritization of a model system
Several research communities have benefitted from the intentional development and promotion of particular model organisms19. One-stop repositories of information such as Flybase, Wormbase, ZFinBase, Xenbase, and Sal-Site have all expanded the research capabilities of participating labs. While many of these labs focus on Developmental Biology, the reach of these model systems includes studies of neuroscience, evolutionary biology, physiology, and ecology. Emulating this deliberate approach will provide vital cohesion and an undoubted boon to investigators studying salamander regeneration and development. While salamanders are a remarkable “model” for regenerative research they are also remarkable organisms for their unique evolutionary histories, ecological roles, life history variation, and conservation biology. Development of tools and resources for the molecular biologists and biochemists working on salamanders will undoubtedly have unintentional spillover benefits into a wider range of research fields.
Outlook
Salamander models continue to be fertile ground for amazing discoveries on regenerative biology, cell differentiation, and development. This conference generated a tremendous amount of motivation for its participants. We have planned a broader 2019 meeting in Massachusetts, which will be a showcase for these recent developments and an iterative checkup on this rapidly growing experimental field.
References
- Reiß, C., Olsson, L. & Hoßfeld, U. The history of the oldest self-sustaining laboratory animal: 150 years of axolotl research. Journal of Experimental Zoology Part B: Molecular and Developmental Evolution 324, 393–404 (2015).
- Wilder, I. W. The Morphology of Amphibian Metamorphosis. (Smith College, 1925).
- In Salamanders in Regeneration Research Methods and Protocols (eds. Kumar, A & Andras, S) (Humana Press, 2015).
- Humphrey, R. R. Albino axolotls from an albino tiger salamander through hybridization. J. Hered. 58, 95–101 (1967).
- Woodcock, M. R. et al. Identification of mutant genes and introgressed tiger salamander DNA in the laboratory axolotl, Ambystoma mexicanum. Scientific Reports 7, (2017).
- Fröbisch, N. B., Bickelmann, C. & Witzmann, F. Early evolution of limb regeneration in tetrapods: evidence from a 300-million-year-old amphibian. Proc. Biol. Sci. 281, 20141550 (2014).
- Elewa, A. et al. Reading and editing the Pleurodeles waltl genome reveals novel features of tetrapod regeneration. Nat Commun 8, 2286 (2017).
- Nowoshilow, S. et al. The axolotl genome and the evolution of key tissue formation regulators. Nature 554, 50–55 (2018).
- Fei, J.-F. et al. CRISPR-mediated genomic deletion of Sox2 in the axolotl shows a requirement in spinal cord neural stem cell amplification during tail regeneration. Stem cell reports 3, 444–459 (2014).
- Flowers, G. P., Timberlake, A. T., McLean, K. C., Monaghan, J. R. & Crews, C. M. Highly efficient targeted mutagenesis in axolotl using Cas9 RNA-guided nuclease. Development (Cambridge, England) 141, 2165–2171 (2014).
- Hayashi, T. & Takeuchi, T. Gene manipulation for regenerative studies using the Iberian ribbed newt, Pleurodeles waltl. Methods Mol. Biol. 1290, 297–305 (2015).
- Nakajima, K., Nakajima, T. & Yaoita, Y. Generation of albino Cynops pyrrhogaster by genomic editing of the tyrosinase Gene. Zool. Sci. 33, 290–294 (2016).
- Fei, J.-F. et al. Efficient gene knockin in axolotl and its use to test the role of satellite cells in limb regeneration. Proc. Natl. Acad. Sci. U.S.A. 114, 12501–12506 (2017).
- Currie, J. D. et al. Live imaging of axolotl digit regeneration reveals spatiotemporal choreography of diverse connective tissue progenitor pools. Dev. Cell 39, 411–423 (2016).
- Khattak, S. et al. Optimized axolotl (Ambystoma mexicanum) husbandry, breeding, metamorphosis, transgenesis and tamoxifen-mediated recombination. Nat Protoc 9, 529–540 (2014).
- Kuo, T.-H. & Whited, J. L. Pseudotyped retroviruses for infecting axolotl. Methods Mol. Biol. 1290, 127–140 (2015).
- Khattak, S. et al. Foamy virus for efficient gene transfer in regeneration studies. BMC Dev. Biol. 13, 17 (2013).
- Oliveira, C. R. et al. Pseudotyped baculovirus is an effective gene expression tool for studying molecular function during axolotl limb regeneration. Dev. Biol. 433, 262–275 (2018).
- Abzhanov, A. et al. Are we there yet? Tracking the development of new model systems. Trends Genet. 24, 353–360 (2008).