Behind the paper: Highlighting skeleton-producing cells during the development of a pentaradial animal
Posted by Heidi Tate, on 24 September 2024
In our recent paper published in Developmental Biology entitled “Localization and origins of juvenile skeletogenic cells in the sea urchin Lytechinus pictus”, we studied the intriguing and unique late larval development of sea urchins with a focus on skeletogenic cells, which deposit the biomineral skeleton. Here, I discuss how this project came about, why we were excited by our findings, some of the challenges faced, and what’s next for the project.
Project beginnings
In 2021, I joined Deirdre Lyons’ lab at Scripps Institution of Oceanography (UC San Diego) to explore my fascination for invertebrate evolution and development. I was excited to develop a project on skeletogenesis and biomineralization in sea urchins, a topic in which Deirdre and I shared interest. As I was new to the field, I dove into the literature and realized that although I was interested in embryonic development, I was most fascinated by the development of the adult body plan, which occurs throughout the late larval stage. This study was also motivated by the development of the sea urchin Lytechinus pictus as a better model species [1-3], which has been an ongoing collaboration between the Lyons lab and the Hamdoun lab, also at UC San Diego. Since L. pictus is a faster developing and more transparent species than other commonly studied sea urchins, we could study and image late larval development in higher detail.
Most sea urchins are indirect developers and undergo a complete switch in body plan during metamorphosis from a bilaterally symmetric swimming larva to a pentaradially symmetric benthic juvenile. Prior to metamorphosis, much of the adult body plan develops on the left side of the larva in a structure called the rudiment, while other smaller adult structures develop in other locations (Fig. 1). I wondered how two drastically different body plans could be made from the same set of genetic information, and how the development of a second body plan could occur outside of a classic embryonic context. I was specifically inspired by a study from 2007 by Mamiko Yajima in which she demonstrated that the juvenile skeletogenic cells arise not from the larval skeletogenic lineage, but from mesodermal cells that had a non-skeletogenic origin in the larva [4], and I found it fascinating that cells with one fate in the larva can be completely reprogrammed to make a new cell type in the adult. I therefore decided to revisit this topic using recent technical advances in the field.
Prior to this study, rudiment development had been described in detail at the tissue level and some immunostaining had been performed [5-9], however mRNA localization via in situ hybridization was challenging due to the thickness and complexity of the late larval tissue. By leveraging HCR RNA-FISH, we were able to conduct some of the first mRNA in situ hybridizations in these late stages as the small probes used in this technique are capable of penetrating into thick late larval tissue. I chose to focus on skeletogenic cells as they have been well-studied during embryonic development of sea urchins for gene regulatory networks and biomineralization [10,11] and had previously been studied in some detail in the late larva [7,8], although not in the newer model species L. pictus. We therefore asked three main questions: where are skeletogenic cells and biomineral located during late larval development in L. pictus within the complex rudiment morphology, what are the most likely origins of the juvenile skeletogenic cells, and are gene expression patterns across skeletogenic cells similar in embryonic and juvenile skeletal development?
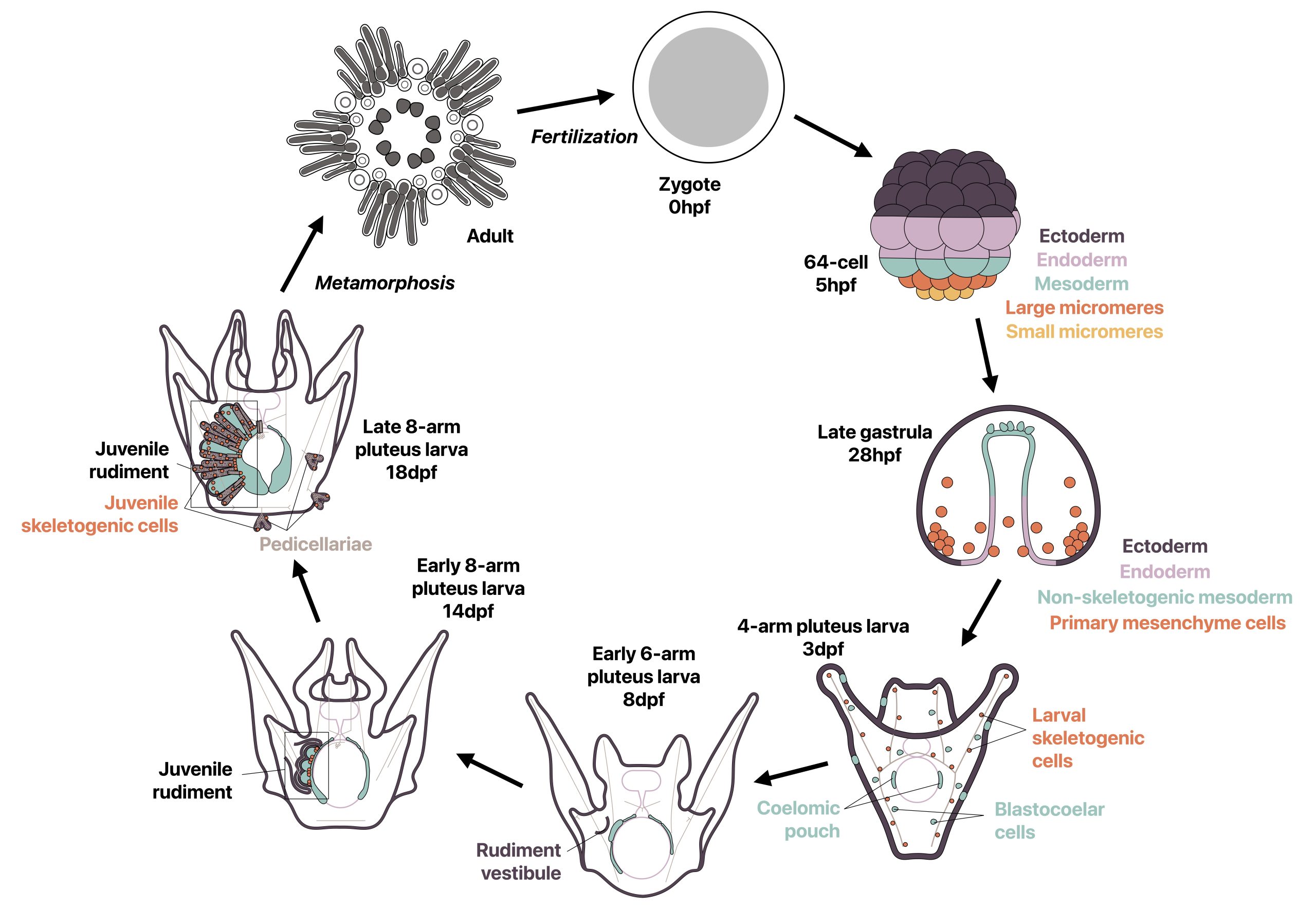
What we learned
We first described in detail the localization of presumptive juvenile skeletogenic cells prior to biomineralization of juvenile skeleton, and at later stages, the localization of biomineral and juvenile skeletogenic cells both within and outside of the rudiment in L. pictus (Fig. 2A-B). We were excited to find that prior to the start of juvenile skeletogenesis in the rudiment, we could identify populations of skeletogenic cells using known biomineral marker genes that were not part of larval rods. Additionally, in some of these presumptive juvenile skeletogenic cells, we found co-expression of our skeletogenic cell marker and a marker of blastocoelar cells, which in the larva act as migratory immune cells (Fig. 2C-D). We therefore proposed that since mesodermal cells of non-skeletogenic origin contribute to juvenile skeletogenic cells [4], that blastocoelar cells may at least in part be the cell type that switches to a skeletogenic fate. We then sampled various biomineralization genes from different gene families and found that mRNA expression patterns throughout juvenile skeletogenesis are similar to the patterns during embryonic development (Fig. 2E-F).
Overall, our work gives insight into how indirect developers, like the sea urchin, can reuse cells and developmental mechanisms to make a new body plan from another. Additionally, it is thought that since all echinoderms have adult skeleton but not all have larval skeleton, that the larval skeleton in sea urchins may be a more recent innovation, while the adult skeletogenic cells could arise from a common origin [12]. Since other echinoderms have blastocoelar-like lineages in the larva [13-14], it is possible that this cell type is the ancestral adult skeletogenic cell lineage that expresses an ancestral skeletogenic gene regulatory network, although this hypothesis needs to be clarified with further work.
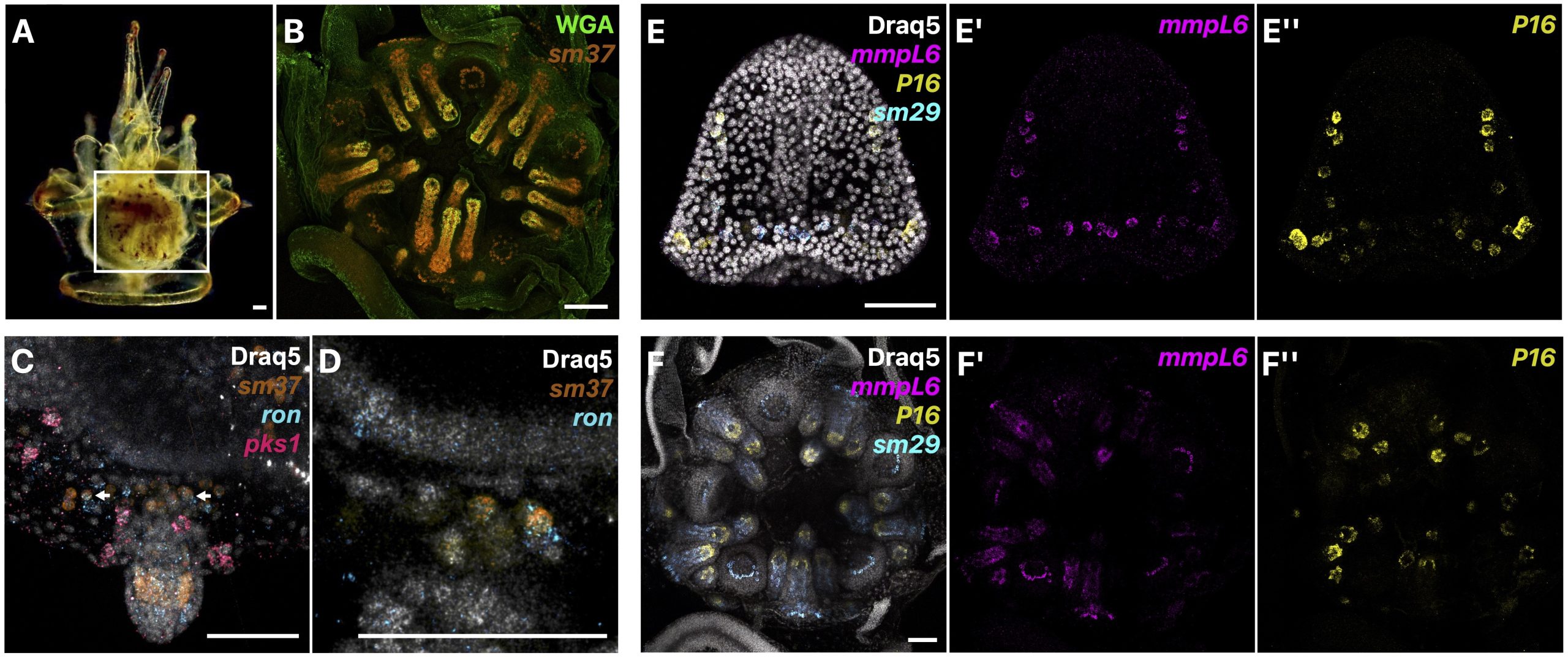
Unexpected challenges
One of the most challenging aspects of this project was identifying a consistent staging scheme during late larval development, which was an important part of the project to help establish L. pictus as a model sea urchin. Interestingly, the larval body plan and parts of the juvenile body plan, like the rudiment, often develop asynchronously, and so similar looking larvae may not have a rudiment at the same developmental stage [5-9]. Additionally, the extent of rudiment development was often different when metamorphosis occurred, leading to post-metamorphic juveniles in different developmental stages. Although frustrating when trying to stage the animals, this helped me think about development in new ways since in indirect developers, adult body plan development may not be as clear-cut as in embryogenesis.
I was also personally challenged to learn about all aspects of the scientific process, from asking clear scientific questions, thinking hard about the best ways to answer them, and learning to tell a compelling story through publication. As with any scientific project, there were many challenges and failed experiments along the way, particularly as I was learning many techniques for the first time. For instance, learning how to mount, orient, and image these large samples added a lot of time to the project, and I often had to retake very long acquisitions when images did not turn out as expected. This really challenged my ability to persevere when experiments were not working, but I felt especially honored and reinvigorated when one of our images was featured on the cover of Developmental Biology. Overall, I was very proud to have finished this work within the relatively short timeline of my master’s degree, and I am greatly looking forward to applying this newfound knowledge in future work.
What’s next with the sea urchins?
With this paper, we highlighted that sea urchin adult body plan development is fascinating, yet understudied, and how it is important to think of body plan development outside of an embryonic context. However, there are still many remaining questions to be answered. For instance, only skeletogenic cells have been studied in some detail during adult body plan development, and HCR RNA-FISH provides the opportunity to understand whole body patterning by investigating other cell types. However, more complex questions, such as mapping where adult cells are originating, would require more complex techniques. With the development of L. pictus as a better system where mature adults can be raised from egg in 4-6 months and with which transgenic lines can be created [1-3], an abundance of new questions could be studied. I am greatly looking forward to continuing work with this amazing animal in the future to answer innovative questions in adult body plan development and evolution. Finally, I am very thankful for my mentors, particularly Dr. Lyons, for the mentorship throughout this project, as well as other collaborators and colleagues who have supported and guided me through past and present research.
References
- Nesbit KT, Fleming T, Batzel G, Pouv A, Rosenblatt HD, Pace DA, Hamdoun A, Lyons DC. 2019. The painted sea urchin, Lytechinus pictus, as a genetically-enabled developmental model. Methods in Cell Biology. 150: 105-123.
- Jackson EW, Romero E, Kling S, Lee Y, Tjeerdema E, Hamdoun A. 2024. Stable germline transgenesis using the Minos Tc1/mariner element in the sea urchin Lytechinus pictus. Development. 151(20).
- Vyas H, Schrankel CS, Espinoza JA, Mitchell KL, Nesbit KT, Jackson E, Chang N, Lee Y, Warner J, Reitzel A, Lyons DC, Hamdoun A. 2022. Generation of a homozygous mutant drug transporter (ABCB1) knockout line in the sea urchin Lytechinus pictus. Development. 149(11).
- Yajima M. 2007. A switch in the cellular basis of skeletogenesis in late-stage sea urchin larvae. Developmental Biology. 307(2): 272-281.
- Cameron RA, Hinegardner RT. 1978. Early events in sea urchin metamorphosis, description, and analysis. Journal of Morphology. 157(1): 21-31.
- Heyland A, Hodin J. 2014. A detailed staging scheme for late larval development in Strongylocentrotus purpuratus focused on readily-visible juvenile structures within the rudiment. BMC Developmental Biology. 14(22): 1-14.
- Yajima M, Kiyomoto M. 2006. Study of larval and adult skeletogenic cells in developing sea urchin larvae. The Biological Bulletin. 211(2): 183-192.
- Smith MM, Smith LC, Cameron RA, Urry LA. 2008. The larval stages of the sea urchin, Strongylocentrotus purpuratus. Journal of Morphology. 269(6): 713-733.
- Formery L, Wakefield A, Gesson M, Toisoul L, Lhomond G, Gilletta L, Lasbleiz R, Schubert M, Croce JC. 2022. Developmental atlas of the indirect-developing sea urchin Paracentrotus lividus: From fertilization to juvenile stages. Frontiers in Cell and Developmental Biology. 10.
- Davidson EH, Rast JP, Oliveri P, Ransick A, Calestani C, Yuh C-H, Minokawa T, Amore G, Hinman V, Arenas-Mena C. 2002. A Provisional Regulatory Gene Network for Specification of Endomesoderm in the Sea Urchin Embryo. Developmental Biology. 246(1): 162-190.
- Wilt FH. 2002. Biomineralization of the Spicules of Sea Urchin Embryos. Zoological Science. 19(3): 253-261.
- Koga H, Morino Y, Wada H. 2014. The echinoderm larval skeleton as a possible model system for experimental evolutionary biology. Genesis. 52(3): 186-192.
- Tamboline CR, Burke RD. 1992. Secondary mesenchyme of the sea urchin embryo: Ontogeny of blastocoelar cells. Journal of Experimental Biology. 262(1): 51-60.
- Metchnikoff E. 1893. Lectures on the Comparative Physiology of Inflammation: Delivered at the Pasteur Institute in 1891.