Diversity is a good thing: coordination of collective cell migration in angiogenesis
Posted by Holly Lovegrove, on 2 February 2017
Comment on “Asymmetric division coordinates collective cell migration in angiogenesis” Nat Cell Bio, 18 (12), 1292-1301, (2016).
Holly E. Lovegrove & Guilherme Costa
Faculty of Biology, Medicine and Health, The University of Manchester, Manchester, Uk
Collective cell migration is involved in many biological processes. In particular it is required to build new tissues during morphogenesis and to repair them during wound healing. Cancer cells however also exploit it during invasion of other tissues. In order for a group of cells to migrate together as a coordinated group they must establish a hierarchy of cellular identities, generally thought of as “leader” and “follower” cells (Friedl & Gilmour 2009). How this hierarchy is established and robustly maintained is key to understanding the process of collective cell migration. To examine this, in our recent study we explored the collective movement of endothelial cells undergoing angiogenesis (the generation of new blood vessels from existing ones) in zebrafish embryos. We were particularly interested in how these collectively migrating cells managed to maintain their organisation while undergoing divisions.
During sprouting angiogenesis the collectively migrating cells take on the roles of either a leading “tip cell” or a following “stalk cell” (Herbert & Stainier, 2011). The tip cell is the first cell to leave the existing vessel and is highly motile. This is then followed by the stalk cells, which are less motile and go on to form the main trunk of the developing vessel. Previous work has established that this hierarchy is driven by differing levels of Vascular endothelial growth factor (VEGF) signalling, with the tip cells having high levels compared to the stalk cells. This is thought to be established via a Notch/Delta controlled lateral inhibition, whereby tip cells induce a reduction in VEGF signalling in the stalk cells (Herbert & Stainier, 2011).
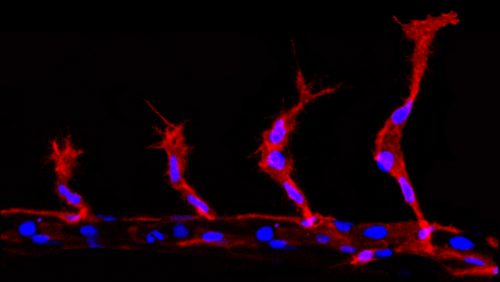
During angiogenic sprouting migrating endothelial cells are required to undergo mitosis (Schoors et al. 2015). This presents the cells with a problem, as the two daughter cells must acquire two different migratory profiles, dependent on their resultant positions. The more distal cell must take on the tip cell identity, while the more proximal cell becomes the trailing stalk cell. However, division will partition the components of the VEGF signalling and Notch pathway components, which if equal will presumably induce competition for tip cell identity between the two daughter cells. This would disrupt the migration of the group of cells and ultimately impede angiogenesis. However, far from this, tip/stalk cell identities are actually established almost instantaneously after division, much faster than notch/delta mediated lateral inhibition is thought to take (upwards of 5 hours) (Matsuda et al. 2015; Bentley et al. 2014).
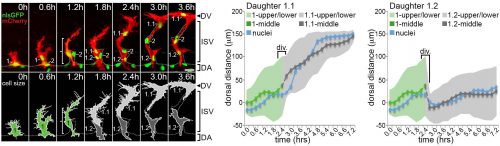
The question – How are the tip/stalk identities of collectively migrating endothelial cells re-established so quickly after mitosis?
Computer modelling suggested that the answer to this question could be that these cells undergo a form of asymmetric cell division and produce daughters of different sizes. The model predicted that a larger daughter cell would inherit more of the VEGF signalling machinery, giving it higher levels of VEGF signalling and thereby establishing it as the tip cell. Live imaging of zebrafish angiogenic endothelial cells revealed that indeed the most distal daughter cell (the tip cell position) is on average 1.8-1.9 times larger than the more proximal daughter cell (the stalk cell position). Furthermore, the size of each daughter cell was shown to be proportional to its migratory speed, meaning that sister cells that had the biggest difference in their size also have the biggest difference in their speeds. Furthermore, in vitro work also demonstrated that larger cells inherit a larger proportion of VEGF receptor mRNA, as well as having higher levels of VEGF signalling. In order to demonstrate that these differing levels of VEGF signalling were necessary to define post-mitotic tip/stalk cell identities, zebrafish embryos were treated with low levels of a drug that blocks VEGF signalling. This low dose didn’t inhibit signalling altogether but prevented any cell from signalling at the levels necessary to be a tip cell. Under these conditions both daughter cells assumed stalk-like identities after mitosis.
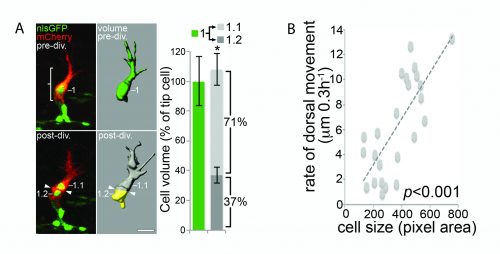
B) The size of a cell is proportional to its speed of migration. Costa et al., (2016)
How might the asymmetry in daughter cell size be achieved?
Preliminary data suggests that the size asymmetry seen in angiogenic endothelial cells is (at least in part) generated by the positioning of the mitotic spindle towards the proximal pole of the cell (Costa et al. 2016). Thereby shifting the division plane away from the volumetric centre of the cell. Other classical asymmetric cell divisions also result in cells of different sizes, for example Drosophila neuroblasts and one-cell C. elegans embryos, though the role of this asymmetry has not been extensively explored (Cabernard et al. 2010; McNally 2013). However some clues may be gleaned as to how angiogenic endothelial cells manage to position their mitotic spindles such that two differently sized daughters are produced. A canonical set of proteins is known to generate the membrane associated pulling force that acts upon mitotic spindles. Partner of Inscuteable (Pins) (LGN in Drosophila and GPR1/2 in C.elegans), anchored at the membrane by Gαi (GOA-1 and GPA-16 in C.elegans), binds to Nuclear mitotic apparatus (NuMA) (Mud in Drosophila and LIN-5 in C.elegans), which in turn binds to the dynein/dynactin complex, this then pulls on the plus ends of astral microtubules (Bergstralh et al. 2013). Asymmetric enrichment of this complex can cause the spindle to be pulled towards one side of the cell, such as in the one-cell C.elegans embryo (Kiyomitsu, 2015). However, it remains to be seen whether the spindle orienting machinery is involved in positioning the mitotic spindle of angiogenic endothelial cells.
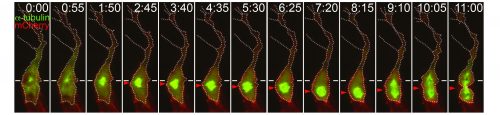
Asymmetric cell division is a well-described phenomenon traditionally thought of as a process employed by cells to enable them to generate cells different from themselves. An asymmetric inheritance of fate determinants or a position dependent asymmetry in external cues normally results in daughter cells becoming two different cells types. Size asymmetry (and a resultant asymmetry in signalling strengths) between daughter cells offers a simple way of introducing subtle heterogeneity into a population of a single cell type. Furthermore, if it is controlled so that the larger and smaller cells are positioned specifically, patterns (such as leading and following cells) can be produced. Further work is needed to elucidate the mechanism behind this new form of asymmetric division and it will be interesting to see whether other collectively migrating systems, or indeed any other cell types, undergo similar divisions.
References
Bentley, K., Harrington, K.I. & Regan, E.R., Can active perception generate bistability? Heterogeneous collective dynamics and vascular patterning. ALIFE http://dx.doi.org/10.7551/978-0-262-32621-6-ch053 (2014)
Bergstralh, D.T., Haack, T. & St Johnston, D., 2013. Epithelial polarity and spindle orientation: intersecting pathways. Philosophical transactions of the Royal Society of London. Series B, Biological sciences, 368(1629), p.20130291.
Cabernard, C., Prehoda, K.E. & Doe, C.Q., 2010. A spindle-independent cleavage furrow positioning pathway. Nature, 467(7311), pp.91–94.
Costa, G. et al., 2016. Asymmetric division coordinates collective cell migration in angiogenesis. Nature cell biology, 18(12), pp.1292–1301.
Friedl, P. & Gilmour, D., 2009. Collective cell migration in morphogenesis, regeneration and cancer. Nature reviews. Molecular cell biology, 10(7), pp.445–457.
Herbert, S.P. & Stainier, D.Y.R., 2011. Molecular control of endothelial cell behaviour during blood vessel morphogenesis. Nature reviews. Molecular cell biology, 12(9), pp.551–564.
Kiyomitsu, T., 2015. Mechanisms of daughter cell-size control during cell division. Trends in cell biology, 25(5), pp.286–295.
Matsuda, M. et al., 2015. Synthetic lateral inhibition governs cell-type bifurcation with robust ratios. Nature communications, 6.
McNally, F.J., 2013. Mechanisms of spindle positioning. Journal of Cell Biology, 200(2), pp.131–140.
Schoors, S. et al., 2015. Fatty acid carbon is essential for dNTP synthesis in endothelial cells. Nature, 520(7546), pp.192–197.