How many and which genes for multicellularity?
Posted by Dictyostelium, on 9 August 2016
My research interest is the evolution of multicellularity. How did cells ‘learn’ to communicate with each other to build a structure that is more complex than its parts and shows new emergent behaviour? Which and how many new genes would be required to transform a unicellular ancestor into a well-organised multicellular structure? The Nature Communications Article from our lab that was published recently (1) sets out to answer some of those questions.
Most of us will be familiar with the type of multicellularity, which evolved in animals, some fungi and plants: cells derived from a zygote continue to divide, but remain attached to each other and eventually differentiate into the different cell types that form the tissues of the organism. All cells are genetically identical and altruistic differentiation into somatic cells that support the propagation of the germline has no cost to them. A quite different case is the colonial multicellularity of social amoebas, which we are studying in our lab. Here, individual cells that can be genetically distinct come together and create a multicellular fruiting structure, consisting of spores and stalk cells. The stalk cells have to altruistically die to support spores. When the amoebae are not genetically identical, conflicts of interest can arise, such as cheating by avoiding stalk cell differentiation. Just like multicellularity by adhesion, colonial multicellularity evolved multiple times (2,3), suggesting that colonial organisms have devised mechanisms to deal with genetic conflict.
The Dictyostelid social amoebas can be subdivided into four major groups, which differ in the size and shape of fruiting bodies, the presence of an intermediate migratory form, the “slug” and the number of cell types, which is largest in group 4. In addition, group 4 species pre-differentiate amoebas into the correct ratio of prespore, prestalk and the other supporting cell types, whereas in groups 1-3 all amoebas first differentiate into prespore cells that then only locally dedifferentiate into stalk cells (Figure 1).
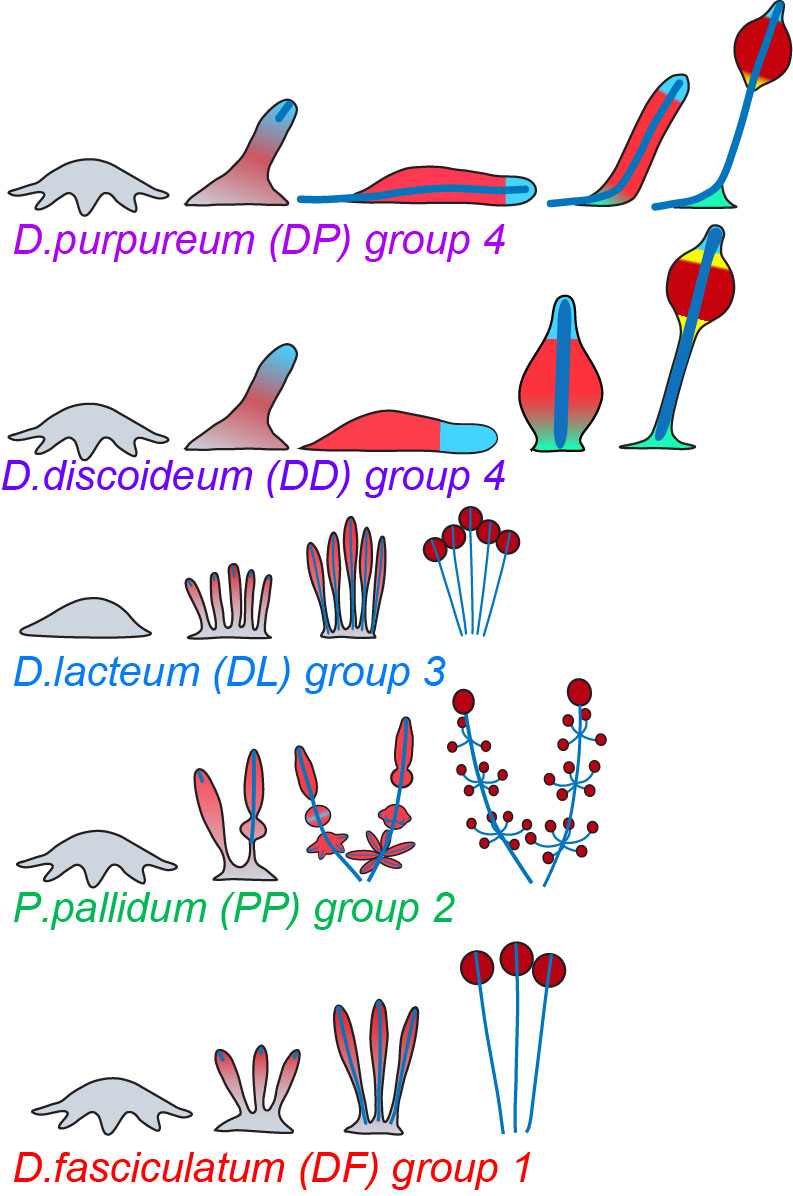
In Dictyostelia, cell proliferation is entirely separated from multicellular development and we can therefore loosely define “multicellularity genes” as genes that are essential for multicellular development, but not for cell proliferation. We wanted to know to what extent such genes were already present in the unicellular ancestors of Dictyostelia and how such genes changed or appeared in the course of Dictyostelid evolution to increase the morphological and behavioural complexity of the organisms. To achieve this we sequenced three genomes that represented groups 1, 2 and 3 of Dictyostelia. The genome of two group 4 species, D. discoideum and D.purpureum were already available as well as the genomes of three unicellular Amoebozoa. Additionally we investigated how expression of all genes in these genomes is regulated during their development by high throughput RNA sequencing. This allowed us to trace gene evolution across the whole phylogenetic tree of social amoebas and their unicellular Amoebozoan relatives. From previous studies, 385 genes in D.discoideum were known to produce a defect in multicellular development when disrupted. We found that 305 of these genes were already present in unicellular Amoebozoa. The majority is conserved in all Dictyostelia regarding the conservation of domains and expression regulation. However, 80% of those genes, which are mainly cytosolic and nuclear proteins and protein kinases, are already present in their unicellular relatives. Eighty genes were unique to Dictyostelia and this set was enriched in plasma membrane and secreted or extracellularly exposed proteins, G-protein coupled receptors and sensor histidine kinases. Also, a set of 37 proteins that were only conserved in group 4 or groups 3 and 4 were highly enriched in plasma membrane and secreted or exposed proteins (Figure 2).
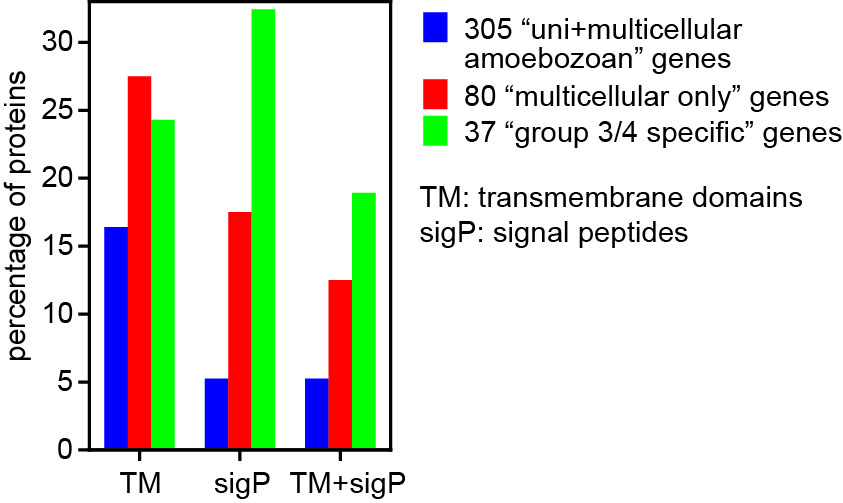
For conserved genes, we also investigated whether their developmental regulation and their protein functional domains were conserved. If not, we scored how such changes were distributed across the Dictyostelium phylogeny. Logically, one expects such changes to be greater when the species are evolutionary more distant from each other. In case of functional domains, the changes were mostly scattered across the phylogeny (Figure 3), suggesting that changes in protein function did not contribute greatly to changes in phenotypic complexity. However, changes in developmental regulation occurred much more frequently between group 4 on one hand and groups 1-3 on the other, than between branches I and II that are evolutionary more distant. Because group 4 species are also phenotypically most distinctive (Figure 1), this indicates that phenotypic innovation in group 4 was more likely to be caused by changes in gene expression than changes in protein function. Finally, investigating the closest relatives of the 385 genes in species outside the Amoebozoa, we found that a relatively large percentage had closest homologs in bacteria. Further scrutiny identified four genes that were only present in Dictyostelia and bacteria and likely entered Dictyostelia by lateral gene transfer. Three of these genes synthesise three out of the five non-peptide signals that induce cell differentiation in D.discoideum: c-di-GMP, DIF-1 and discadenine (4-7).
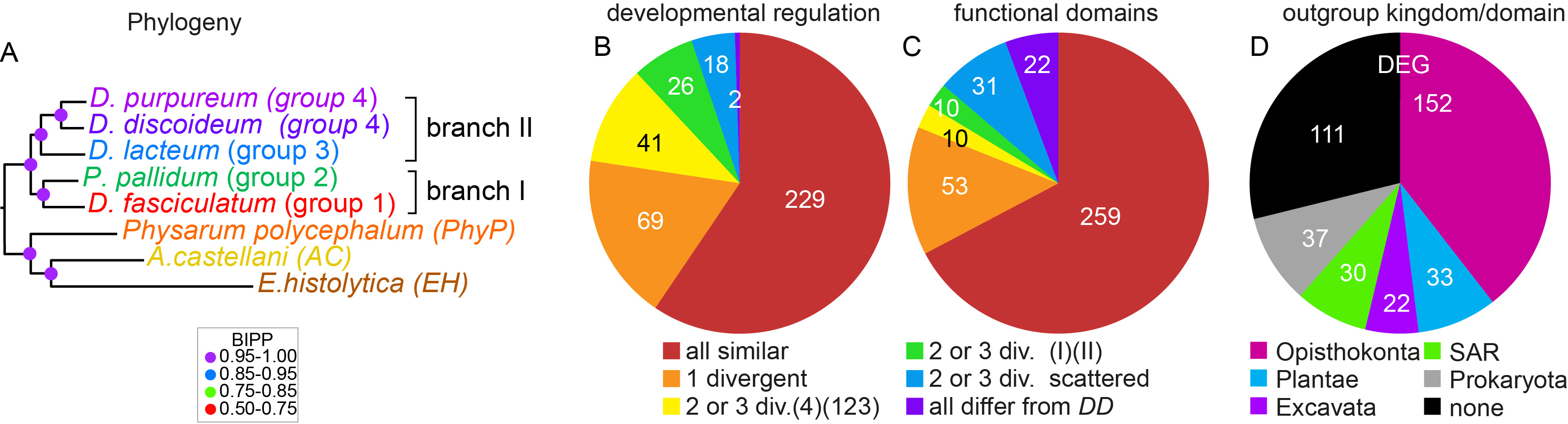
In conclusion, it seems that innovation to multicellularity largely relied on repurposing of existing genes that were already present in the unicellular ancestor. Conversely, genes encoding exposed and secreted proteins with likely roles in adhesion and cell communication and the sensors to detect these signals appeared only in the multicellular forms, with genes for some novel signal molecules being acquired directly from bacteria. Furthermore, changes in gene regulation appear to have been more important for evolution of phenotypic complexity than changes in gene function.
References:
- Glockner, G., et al., The multicellularity genes of dictyostelid social amoebas. Nature communications, 2016. 7: p. 12085.
- Du, Q., et al., The Evolution of Aggregative Multicellularity and Cell-Cell Communication in the Dictyostelia. Journal of molecular biology, 2015. 427(23): p. 3722-33.
- Schilde, C. and P. Schaap, The Amoebozoa. Methods in molecular biology, 2013. 983: p. 1-15.
- Abe, H., et al., Structure of discadenine, a spore germination inhibitor from the cellular slime mold. Tetrahedron Letters, 1976. 17(42): p. 3807-3810.
- Chen, Z.H. and P. Schaap, The prokaryote messenger c-di-GMP triggers stalk cell differentiation in Dictyostelium. Nature, 2012. 488(7413): p. 680-3.
- Neumann, C.S., C.T. Walsh, and R.R. Kay, A flavin-dependent halogenase catalyzes the chlorination step in the biosynthesis of Dictyostelium differentiation-inducing factor 1. Proceedings of the National Academy of Sciences of the United States of America, 2010. 107(13): p. 5798-803.
- Saito, T., A. Kato, and R.R. Kay, DIF-1 induces the basal disc of the Dictyostelium fruiting body. Developmental biology, 2008. 317(2): p. 444-53.