Mechanical control of the inner ear development
Posted by kgnedeva, on 14 September 2017
Prologue
Molecular biological approaches to understanding the complex gene networks regulating development often resemble attempts to understand a Monet painting by looking at it up close. We find the links within a disorganized array of brush strokes and shapes but may miss the big picture. Our recent study is an example of how one must sometimes take few steps back to admire the water lilies.
Why do we study inner ear development?
The subject of our investigation is a vestibular sensory organ—the utricle (Fig. 1). Our ability to perceive accelerations and to orient ourselves in three-dimensional space relies upon the operation of this and four other vestibular organs within the inner ear (Utricle and Saccule balance and equilibrium). Despite the differences in their functions, the vestibular organs as well as the hearing organ, or cochlea, are populated by the same type of mechanosensitive receptors—the hair cells. Mammals develop a specific number of these cells during embryonic development; no additional hair cells are formed throughout life, and once lost, these sensory receptors cannot be restored naturally. Hair cells are vulnerable to many environmental and health hazards that are prevalent in industrialized countries: loud noises, certain antibiotics, and anticancer drugs. As a result, deficiencies of hearing and balance often occur owing to the irreversible loss of hair cells. Regeneration of the sensory receptors represents a promising approach for treating these disorders. However, understanding the molecular basis of sensory organ development is a necessary first step towards making this treatment a reality.
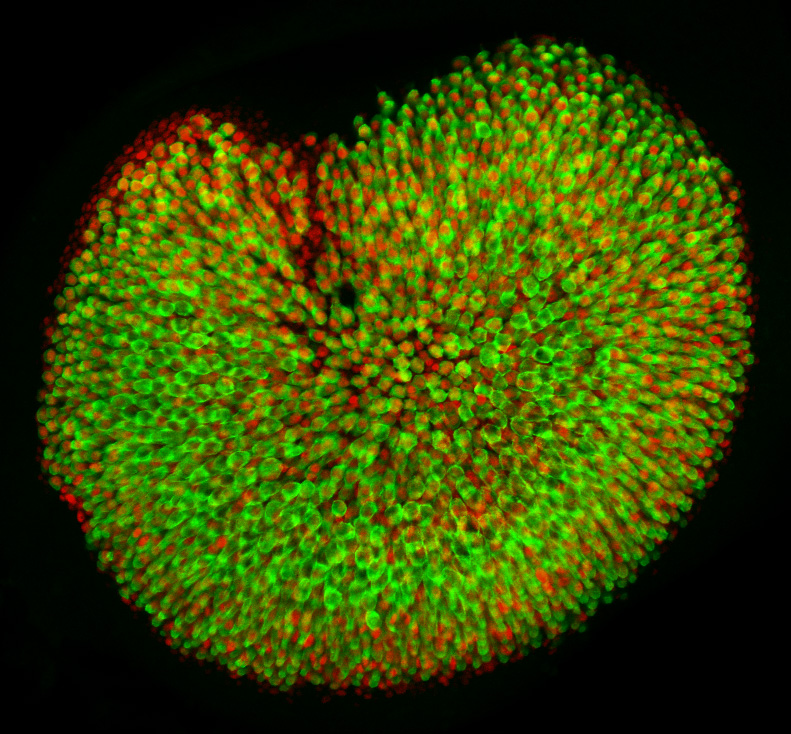
The original idea for our work
The size of each of the inner ear sensory organs, such as the utricle, shows miniscule variation, suggesting a tight control of growth. Whereas many molecular pathways that stimulate sensory cell proliferation during early development have been identified, the growth control mechanism that arrests proliferation in these organs has not been understood. By combining computational modeling and molecular biology we sought to investigate this mechanism in the utricle (Gnedeva et al., 2017).
Instead of looking first at the molecular, “up close” level by investigating the gene-expression changes accompanying development of the utricle, we decided to take a few steps back and to apply a macroscopic, engineering approach to understand the mechanisms controlling its growth. By measuring the changes in area of the utricle during development starting at embryonic day 15.5 (E15.5), we found that the organ’s growth slows as the utricle approaches its final size by postnatal day 2 (P2) (Fig. 2). In other words, the rate of the organ’s growth correlates negatively with its size. This observation suggested a self-regulatory mechanism of epithelial growth and implied a negative-feedback mechanism—a previously unexplored possibility of growth control in sensory organs of the inner ear.
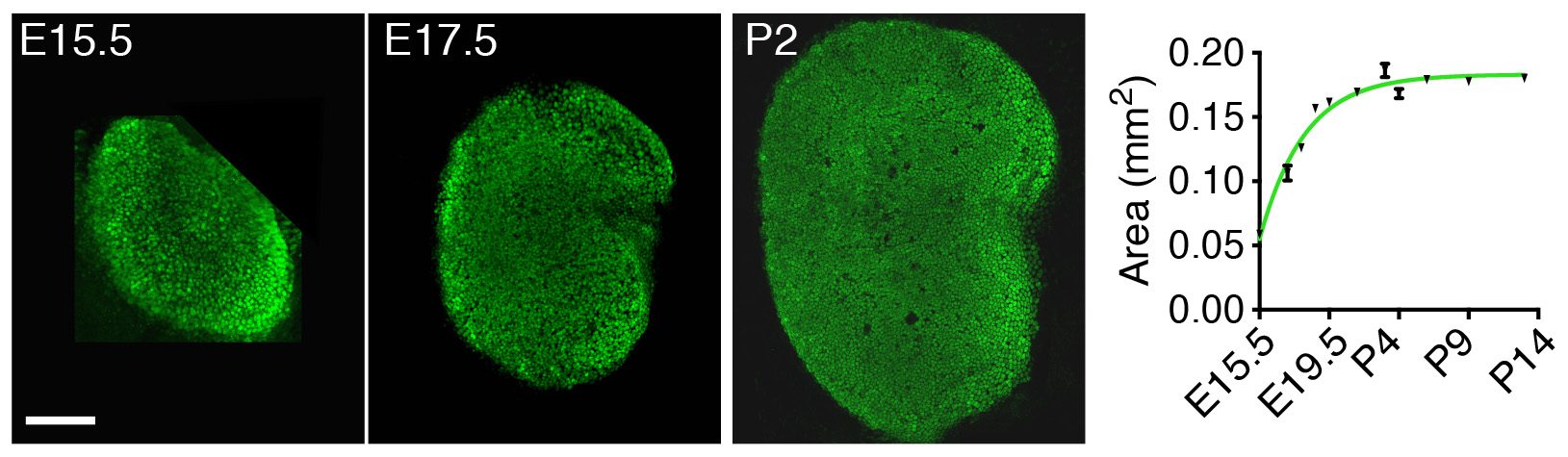
Surprisingly, in biological systems only two major self-regulatory negative feedback mechanisms are known to regulate organ growth. The first was discovered when in vitro studies demonstrated that many cell types produce morphogens that suppress their own proliferation. One of the first examples of such molecules is a member of the TGF-β family of morphogens—myostatin, which is produced by skeletal muscles to inhibit their growth. Many homologous molecules have since been discovered to control growth in various other tissues.
The second mechanism of growth control is related to mechanical constraints. It has long been observed that cells cease to proliferate in culture when they fill up the available space. The molecular basis of this phenomenon, called contact inhibition of cell growth, has recently been discovered. In brief, cell-cell contacts, as well as changes in pressure and tissue stiffness, can activate the Hippo signaling pathway. The Hippo kinase cascade subsequently targets the transcriptional cofactors Yap and Taz, resulting in their degradation; this in turn inhibits cell growth and proliferation.
In our work we created morphogen-limited and elasticity-limited computational models to incorporate each of the two growth control mechanisms and to explore which one of them is more dominant in regulating cell proliferation in the utricle.
What did we discover?
Because the models included the negative-feedback control of growth, they could both accurately reproduce the observed pattern of utricular area expansion. To distinguish between the models, we developed an ex vivo culture system that allowed for manipulation of the elastic force independently of the morphogen concentration in the system.
We found that reducing the mechanical constraints normally present during development led to a striking expansion of utricular area (Fig. 3). This demonstration that elastic force retards the growth of the utricle suggested the involvement of the Hippo signaling cascade. Our experimental data confirmed this prediction: the excessive growth observed in low-stiffness conditions was triggered by the accumulation and nuclear translocation of Yap protein—the direct target of Hippo signaling (Fig. 3).
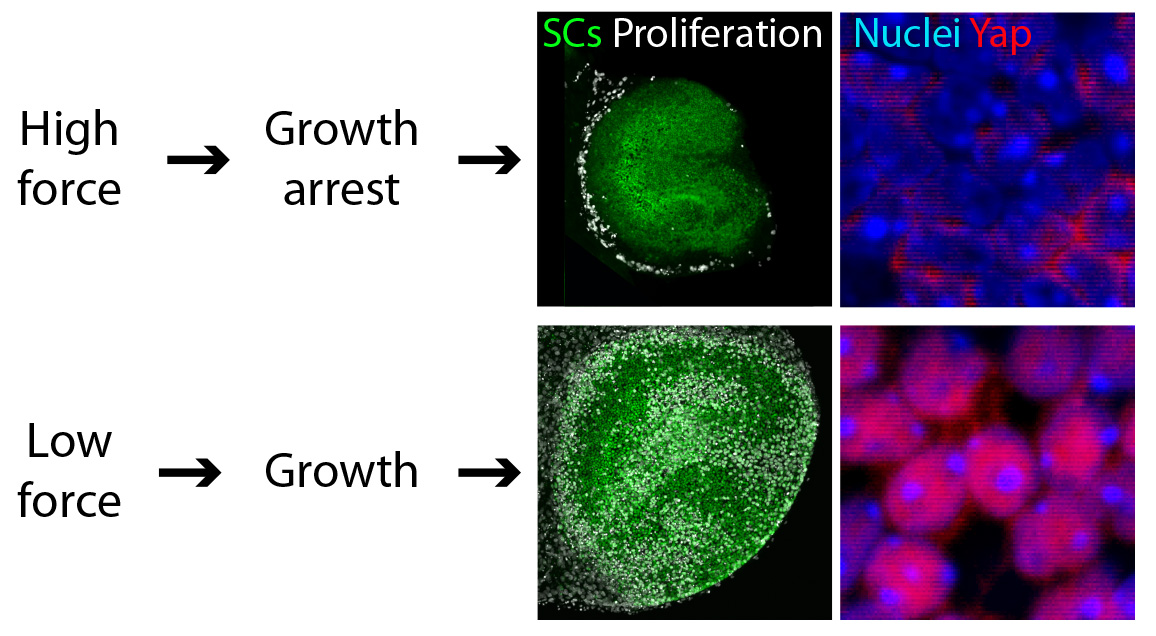
We confirmed that the pattern of cell proliferation normally observed in the developing utricle can be explained by the distribution of the forces in the tissue, as predicted by the model, and accords with the expression pattern of Yap protein. Importantly, we also found that the role of Yap is not limited to development; nuclear Yap translocation is also induced during the limited regeneration observed in the utricle. Because cell proliferation is a first step necessary for recovery of the inner ear sensory functions, we predict that altering Hippo signaling might induce regeneration. The strength of this approach, as opposed to gene therapy, is that the kinases in the Hippo pathway can be targeted pharmacologically. Although further investigation of the role of Hippo signaling in the inner ear is required, biochemical manipulation of this pathway might aid in the recovery of hearing and balance after the loss of hair cells.
In conclusion
Methodological advances during the past few decades have progressively specialized and separated the branches of biological research. Although this specialization has led to great progress in our understanding of living matter at a deeper level, the separation has come at a cost. It is increasingly clear that a cross-disciplinary approach can restore a broader perspective and facilitate the investigation of complex biological systems. Our work constitutes another example of the benefits of such an approach. A combination of mathematical and biological methodologies has allowed us to uncover a growth control mechanism regulating the development of sensory epithelia in the inner ear. Our hope is that building upon our original model, for example by adding the processes of cell differentiation and cell death, can yield an understanding of other aspects of inner ear development, such as the mechanism of pattern formation and regeneration in the system.
All the illustrations are adopted from the original article:
Gnedeva K.*, Jacobo A.*, Salvi J., Petelski A., and Hudspeth A. J. Elastic force restricts the growth of the murine utricle. eLife in press (2017). DOI: 10.7554/eLife.25681
* These authors contributed equally to the manuscript