Patterning in scale: a lesson from avian embryos
Posted by aysuygur, on 7 July 2016
A fundamental property of all organisms is the remarkable consistency within the formation of the body plan during development. A handful of conserved molecular pathways are responsible for morphological patterning in embryos across different taxa, despite an enormous diversity of embryonic size in nature. Remarkably, morphological patterns are scaled to tissue size at the time of development and same structures are maintained in relative proportions to the overall size.
One classic mechanism of pattern formation is the morphogen model, where a molecule – the morphogen – is secreted from cells at one end of a developmental field, and diffuses into the target tissue (Lander 2007). At any given time point, morphogen concentration is different around cells that are closer versus further away from the morphogen source. The target tissue responds by activating distinct transcriptional programs in progenitor domains that are exposed to different morphogen concentrations, leading to establishment of different cell fates along the length of the tissue. Therefore, size of the target tissue is an integral component of the patterning process.
How is the morphogen mechanism adjusted, then, to trigger the same transcriptional responses in proportional domains across tissues of different sizes in closely related species? In many instances, morphological patterns are set when tissues size is similar: human embryos are roughly the same size during embryogenesis, and differential growth can account for why taller and smaller human individuals nevertheless share similar body proportions. However, in other cases members of related taxa have quite different sizes during embryonic development, when the morphological pattern is being established. How conserved pathways that regulate patterning are modified to generate a size-invariant output is a biological problem that still remains to be solved (Figure 1).
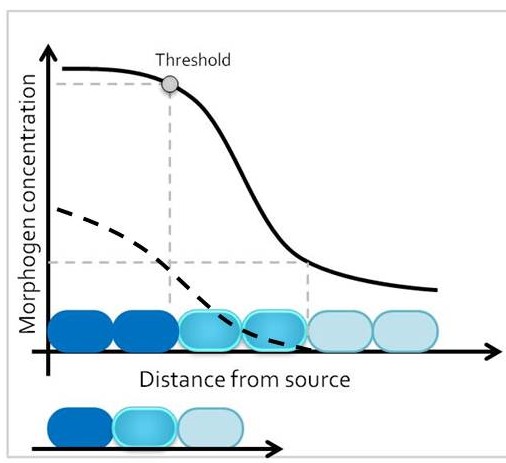
In our recent paper from the Tabin lab, we tackled this problem through the study of aves, a clad of organisms that show a great variation in size – think of the range of egg sizes from an ostrich egg to a hummingbird egg (Uygur et al. 2016). Yet, these animals are related close enough that we would expect patterning mechanisms to be highly conserved. This gave us the flexibility to study how scaling of the body plan is achieved across two organisms with a drastic difference in embryonic size, the chick (Gallus gallus) and the tiny zebra finch (Taeniopygia guttata) (Figure 2). The neural tube provided to be an excellent model system to explore this question in aves. Pioneering studies in the past two decades have shown that the sonic hedgehog (SHH) morphogen secreted from the notochord and floorplate induces distinct transcriptional programs and cell fates at different concentrations along the ventral half of the neural tube (Liem et al. 2000; Dessaud et al. 2007; Stamataki et al. 2005). As is the case with the morphogen model systems, tissue size and distance from source is critical to patterning onset in this context.
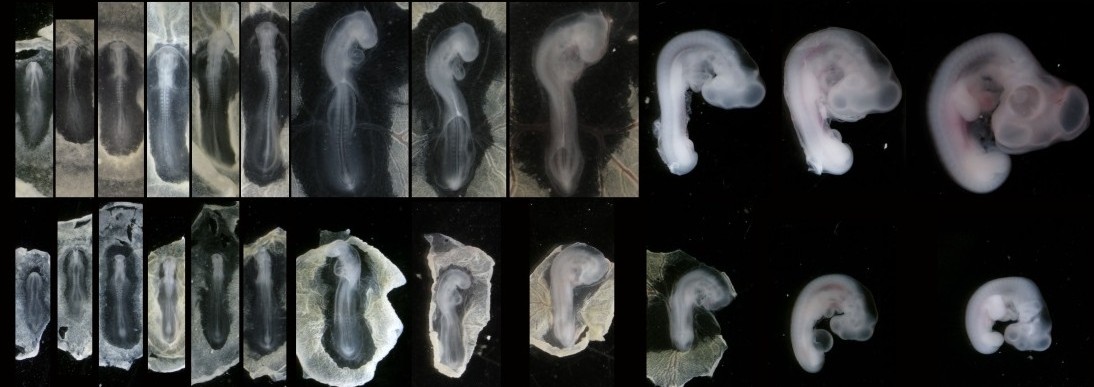
When we examined the establishment of pattern in the ventral neural tube of chick versus zebra finch embryos, we were surprised to find out that it is not only the tissue size that varies between the two species, but also the duration of the patterning process. Across highly similar developmental stages and timepoints, the zebra finch ventral neural tube is patterned much faster compared to the chick neural tube. However, when we compare the levels of morphogen secretion, we found out that the zebra finch notochord, which is drastically smaller than the chick notochord, is also generating a morphogen gradient of much lower amplitude throughout development. Even though the smaller species is producing less morphogen, it is patterning the tissue at a much faster rate. What is going on?
In the past, adjustments to the morphogen mediated patterning systems have been explored at the level of adjusting the morphogen gradient itself(Cheung et al. 2014; Gregor et al. 2005; Ben-Zvi & Barkai 2010). Even though this may still be an interesting aspect of the scaling of the neural tube patterning, our findings lead us to explore differences in morphogen responsiveness. According to our initial observations, patterning process in the smaller zebra finch tissue is completed much faster, with less morphogen in the tissue. When exposed to the same concentration of the sonic hedgehog morphogen, would cells from the zebra finch versus chick turn on the same transcriptional program and cell fates? We turned back to classical embryology to answer this question and generated chimeric embryos at a very early time point in development (at 12 hours, to be exact). By transplanting cells between transgenic lines from the two species, we generated embryos with chimeric neural tubes that were made up of both zebra finch and chick cells, where cells from the two species were exposed to the same developmental signals and received the same level of SHH morphogen from a single source. Remarkably, we observed zebra finch cells to be much more sensitive to the morphogen compared to adjacent chick cells that are same distance away from the source. An analysis on the expression of different transcription factors confirmed that the transcriptional profile of the zebra finch cells were of a higher level morphogen threshold, as if they had seen a higher concentration of SHH for longer durations. This shows cell autonomous differences in morphogen sensitivity account for differential response to the morphogen and altered rates of patterning (Figure 3).
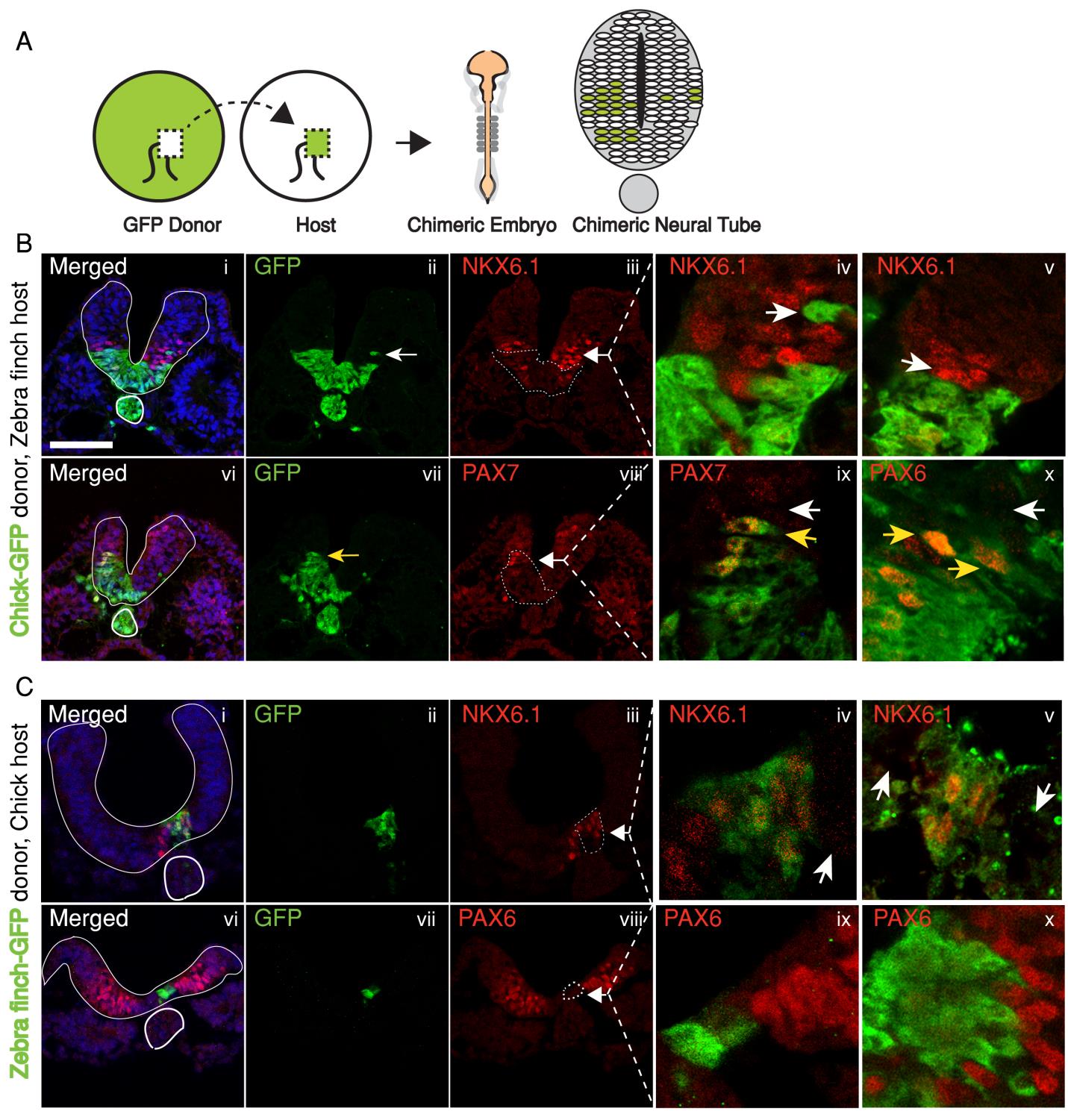
The strength of using the neural tube as a model is that the readout for morphogen activity is very well characterized in this system. We took advantage of this to quantify the differential response of neural progenitors to Sonic hedgehog in the two species. In an explant in-vitro culture system, we analyzed response to different concentrations and durations of SHH in neural tube naive explants isolated from either zebra finch or chick embryos. Indeed, all morphogen thresholds that have previously been identified for chick cells were lower for zebra finch cells, meaning that the zebra finch tissue can induce same cell types at lower concentrations and lower exposure durations.
Our studies on the mechanism of this difference showed that the differential response persists even when the SHH pathway is activated directly through its Smoothened receptor via an agonist. This finding revealed that the cell autonomous differential response is an intra-cellular difference downstream of the patched and smoothend receptors. We further showed that differential levels of the sonic hedgehog transcriptional effortors may be responsible for the differential response, as the two species display different levels of the GLI3 transcript. When we manipulated the zebra finch embryos to express higher levels of GLI3, we observed the speed of patterning is altered and the domain ratios are not scaled to the smaller tissue size.
Our study offers a potential explanation to how tissues of different scales achieve proportional domain sizes when using the same signaling pathways for patterning. It appears that in the case of chick and zebra finch, two avian embryos of different sizes, cell intrinsic differences in morphogen sensitivity control scaling of cell fate patterns in the ventral neural tube. We hope this work will provide new venues to study pattern scaling in order to enhance our understanding of how evolutionary mechanisms accommodate adjustments to body size.
Ben-Zvi, D. & Barkai, N., 2010. Scaling of morphogen gradients by an expansion-repression integral feedback control. Proceedings of the National Academy of Sciences of the United States of America, 107(15), pp.6924–9. Available at: http://www.pubmedcentral.nih.gov/articlerender.fcgi?artid=2872437&tool=pmcentrez&rendertype=abstract [Accessed July 11, 2014].
Cheung, D. et al., 2014. Adaptation of the length scale and amplitude of the Bicoid gradient profile to achieve robust patterning in abnormally large Drosophila melanogaster embryos. Development (Cambridge, England), 141(1), pp.124–35. Available at: http://www.ncbi.nlm.nih.gov/pubmed/24284208 [Accessed April 12, 2014].
Dessaud, E. et al., 2007. Interpretation of the sonic hedgehog morphogen gradient by a temporal adaptation mechanism. Nature, 450(7170), pp.717–20. Available at: http://www.ncbi.nlm.nih.gov/pubmed/18046410 [Accessed March 21, 2014].
Gregor, T. et al., 2005. Diffusion and scaling during early embryonic pattern formation. Proceedings of the National Academy of Sciences of the United States of America, 102(51), pp.18403–7. Available at: http://www.pubmedcentral.nih.gov/articlerender.fcgi?artid=1311912&tool=pmcentrez&rendertype=abstract [Accessed March 26, 2014].
Lander, A.D., 2007. Morpheus unbound: reimagining the morphogen gradient. Cell, 128(2), pp.245–56. Available at: http://www.sciencedirect.com/science/article/pii/S0092867407000517 [Accessed December 10, 2014].
Liem, K.F., Jessell, T.M. & Briscoe, J., 2000. Regulation of the neural patterning activity of sonic hedgehog by secreted BMP inhibitors expressed by notochord and somites. Development (Cambridge, England), 127(22), pp.4855–66. Available at: http://www.ncbi.nlm.nih.gov/pubmed/11044400 [Accessed August 12, 2014].
Stamataki, D. et al., 2005. A gradient of Gli activity mediates graded Sonic Hedgehog signaling in the neural tube. , pp.626–641.
Uygur, A. et al., 2016. Scaling Pattern to Variations in Size during Development of the Vertebrate Neural Tube. Developmental Cell, 37(2), pp.127–135.
This is perhaps one of the smartest molecular, embryo logical and comparative demonstrations I am aware of how cell autonomy may regulate species-dependent embryonic heterochronies. Understanding how the amplification of the SHH signal is accomplished in finch embryonic cells may shed light on the molecular processes targeted by evolution and environmental pressures to generate diverse allometries between closely and even distantly related organisms.