Tensed beginnings: how membrane tension gates early differentiation
Posted by Henry De Belly, on 9 June 2021
Mouse Embryonic Stem Cells (ES cells) have the capacity to generate any tissue in the organism; this remarkable ability is called naïve pluripotency. Intriguingly, when ES cells start to differentiate they undergo a striking shape change.
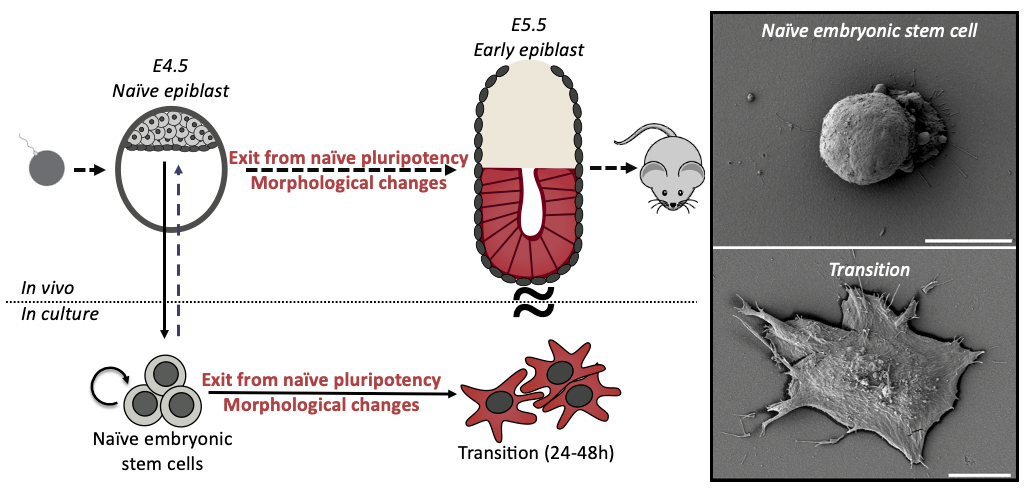
During my interview with Ewa (Paluch), my PhD supervisor, we were discussing potential projects for me, when she showed me some preliminary scanning electron microscopy pictures of differentiating ES cells. I was immediately struck by the idea of fate changes coupled to cell shape changes. ES cells are round and smooth, whilst differentiating cells are spread with many membrane folds. As cell shape is dictated by cell mechanics, this shape change suggested a profound mechanical change during differentiation. We wondered if the shape change was a consequence of the fate transition, or was it a cause? This sounded like a great question to investigate for a PhD, at the interface between two cool labs (Ewa Paluch and Kevin Chalut) and so a few months later I joined, ready to dive into mechanics of cell fate transitions.
When observing ES cell fate transition in more detail, we noticed cells blebbing (blebs are pressure-driven spherical protrusions) intensely before spreading. This was pretty interesting because such blebbing is often a sign of changes in the organisation of the actin cytoskeleton, and notably membrane to cortex attachment. We measured this attachment during early differentiation (using microscopy & Western Blots) and found striking differences between naïve and differentiated cells. This was an important result for us as we knew from the literature that membrane to cortex attachment is the main regulator of effective membrane tension, which has been shown to play an important role in regulating cell shape and function.
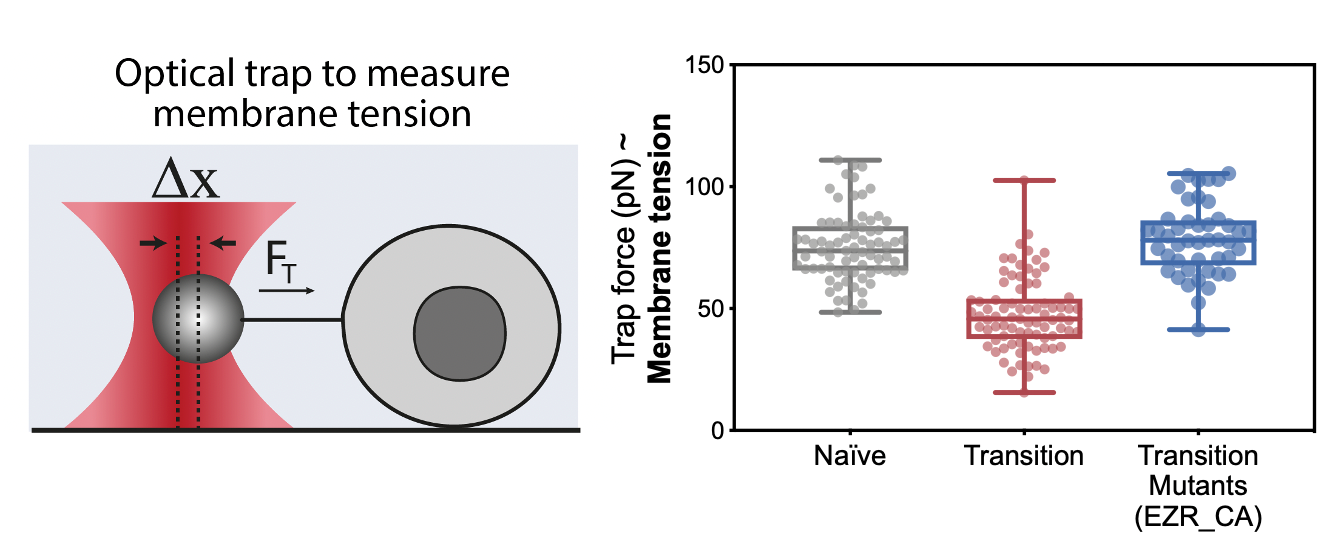
The next step was to measure membrane tension during early differentiation; however, we didn’t have the setup ready for it just yet. In this frustrating interregnum, we decided to push ahead anyway and to try mutants and drugs that should generate defects in membrane tension. These preliminary experiments turned out to be a key milestone of this project: drugs and constructs increasing membrane tension led to major differentiation defects.
By the time we got these results, we had set up our system to measure membrane tension: a tether pulling assay using an optical trap. This technique is great because it enables precise membrane tension measurements without disturbing cell shape. As we hypothesized, we found that as cells differentiate, they undergo a dramatic drop in membrane tension. Furthermore, we were able to verify our membrane tension mutants, and establish that maintaining high membrane tension during fate transition (using drugs or mutants) leads to significant early differentiation defects. We also used our mutant cell line to show that maintaining high membrane tension resulted in developmental defects in gastruloids and cultured embryos.
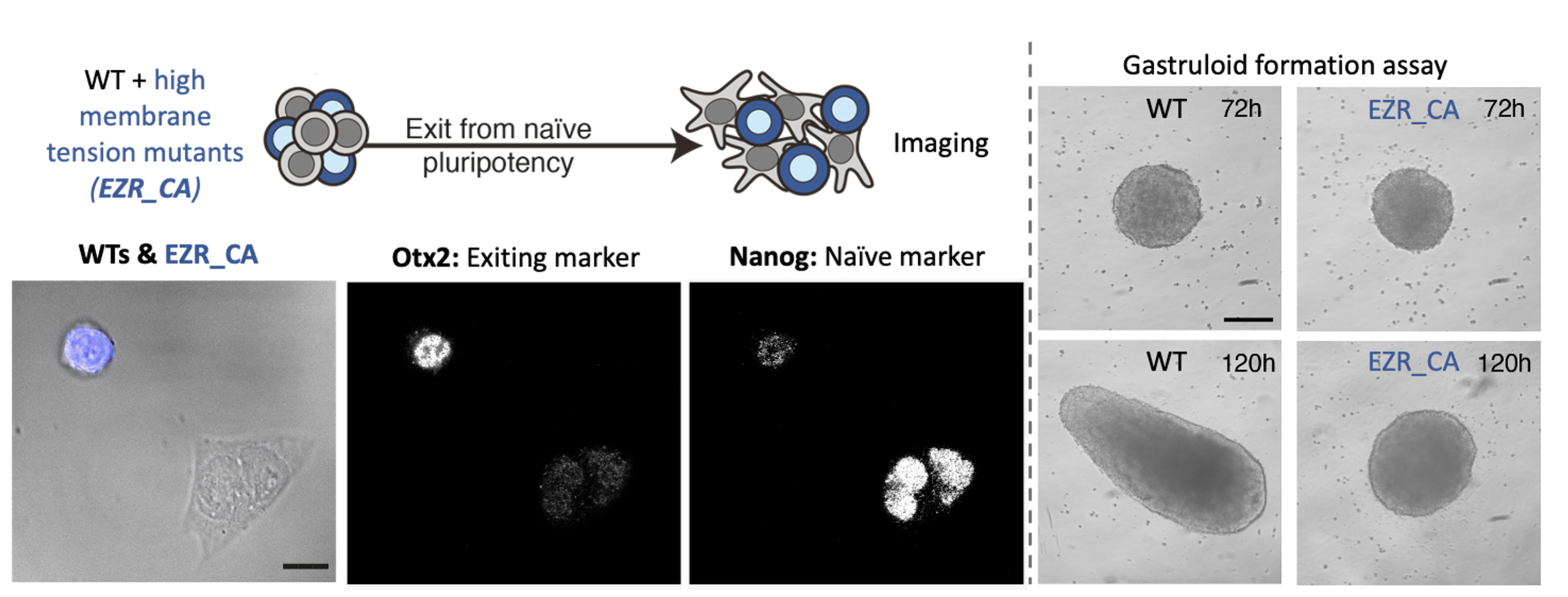
The next goal was to identify a mechanism by which changes in membrane tension could regulate differentiation. We tried to perturb various mechanosensors to no avail until one day Kevin had the idea of looking at endocytosis. Endocytosis is negatively regulated by membrane tension and is also a major regulator of signalling. We measured endocytosis in ES cells and found that it increases sharply as cells decrease their membrane tension and change shape. We next figured out a way to increase endocytosis levels in cells with high membrane tension (mutants) and found that this was sufficient to rescue their differentiation defects. At that stage we knew endocytosis was downstream of membrane tension but what was downstream of endocytosis? In other words, what was the link between increase in endocytosis and ES fate transition?
We decided to look at ERK activation, because ERK activation is required for early differentiation and recent work by the Scita lab beautifully showed that ERK is, in part, activated in the early endosome (Palamidessi et al., 2019). The Scita lab kindly provided us with a FRET sensor which allow to measure ERK activity in early endosome. We found that ERK activity sharply increased in cells as soon as they spread, which correspond to their drop in membrane tension and increase in endocytosis.
When we put the story together for preprint, we agreed that the main missing bit of the story was that we didn’t know what was triggering the observed change in membrane tension. After a few exploratory experiments we found out that this drop in membrane tension was triggered by Beta-catenin degradation. This degradation is coupled to a significant drop in RhoA activity, which is a known regulator of cell mechanics and notably membrane tension. This result was pretty incredible because it tied together two key pluripotency pathways, beta-catenin and ERK, via an intrinsic change in cell mechanics (membrane tension).
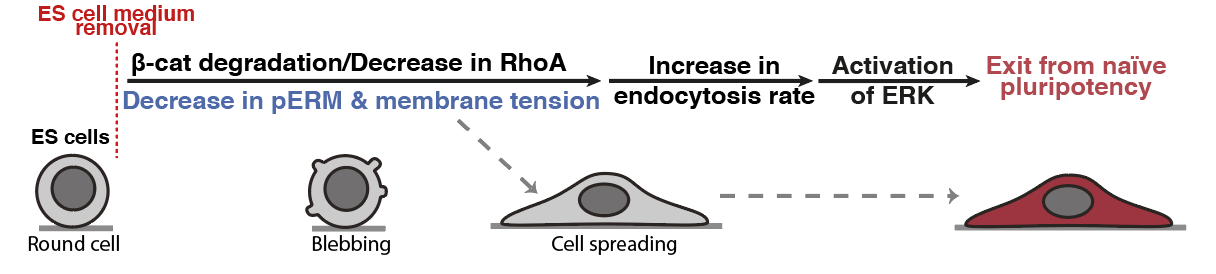
Figure 4: Membrane tension gates early differentiation. (Belly et al., 2021)
One aspect I really like about this paper is that it highlights the role of intrinsic cell mechanics in regulating cell mechanics. Before working on this, I always considered the role of mechanics from the environment point of view, for example how different substrate stiffnesses could regulate fate. But here we could show that cells can intrinsically modulate their mechanical properties, independently of their environment, and in turn regulate signalling. This change is clearly tied to changes in cell state, and speaks to another level on which cells tune their own receptiveness to signalling.