When Two Make One: The Uses and Importance of Plant Grafting
Posted by cmelnyk, on 29 December 2015
Used for thousands of years but grafting remains mysterious
For millennia, people have cut and joined different plant varieties or species together by a process known as grafting. By grafting different plants to each other, the chimeric individual acquired desirable properties from both plants, or alternatively, elite varieties could be more easily propagated. It is unknown how people first discovered that different plants could be grafted together, but nature may have provided inspiration since certain plants naturally graft with themselves (such as English Ivy) and occasionally, to other species. The first uses for grafting were likely to propagate desirable varieties of fruits such as oranges, apples, plums, cherries, grapes, figs and olives [1] since the best varieties are often not true breeding (the seeds will not produce fruit identical to the parents). Later, improving plant size or improving disease resistance may have encouraged further widespread use of this technique. A great example of this phenomenon is the collapse of the European wine industry in the 1880s due to a flying insect, phylloxera, which had arrived from North America. Similar to an aphid, it fed on the leaves and roots of European grapes that had no immunity, unlike North American grapes. The solution was to graft a North American rootstock to a European scion (shoot), thus conveying resistance to the roots where the majority of the damage occurs. Grape grafting spread throughout Europe, the Americas, Oceania and the industry was saved. Today, the vast majority of vineyards, orchards and many ornamental plants and vegetables are grafted for disease resistance, ease of propagation or to change plant size.
People have been interested in how plants graft for at least several hundred years. Texts published in the 1500’s and 1600’s described how to graft and which species could be grafted. Interestingly, much of the compatibility information was incorrect [1], and it took several centuries before a more rigourous scientific approach was taken. John Wright performed some of the first studies in the 1890s when he described the events that occurred during grafting between tomatoes, potatoes and geraniums [2]. Later, using scanning electronic microscopes, breaking weight assays and sectioning and staining for cell division and vascular connectivity shed light on the grafting process [3, 4]. These studies described a process whereby tissues initially adhere, followed by undifferentiated tissue formation (callus), and finally by vascular differentiation and reconnection [4, 5]. Cell wall components including pectins were deposited [4], and cytoplasmic channels formed between the two grafted halves [6, 7]. Despite these comprehensive descriptions, we had little idea of the mechanism or developmental process that allows plants to graft.
Arabidopsis as a way forward
The majority of previous grafting studies used commercially important species such as grapes and tomatoes but their limited genetic resources made research with these species slow and labour intensive. One solution was to use Arabidopsis, the model plant. Fortunately, an efficient and rapid protocol for grafting Arabidopsis had been developed [8] and used extensively to study the movement of proteins, RNAs and metabolites [9]. We embarked in early 2012 to study how the Arabidopsis hypocotyl (the tissue between the shoot and root) reconnects, the results of which have been published recently [10]. Initially, we focused on the vascular reconnection process since previous work indicated that this was a critical step for successful graft formation [5]. The technique involved grafting seven-day-old seedlings and initially we used attachment assays and transport assays to look for the movement of fluorescent dyes and fluorescent proteins (GFP) across the graft junction. We observed a dynamic process involving attachment of cut tissues, followed by phloem connection, then root growth resumed and finally xylem connection. We performed live imaging and in situ hybridisations of the grafted tissues and observed cell division and cell differentiation occurring first above the junction (scion side) and one to two days later, immediately below the junction (rootstock side). Promoters responsive to the plant hormones auxin and cytokinin were also strongly unregulated in the region of the graft. The functional assays using GFP transport were robust and reproducible which facilitated a reverse genetics approach. Since plant hormones responses were increase and these hormones are known to be important for vascular formation, we assayed 45 genotypes associated with the auxin, cytokinin and ethylene pathways and found four genotypes, all in the auxin response pathway, were important for phloem connection. We further analysed two of these mutants (alf4 and axr1) and, surprisingly, found they were only required below the graft junction and only in the region close to the cut site (Figure 1).
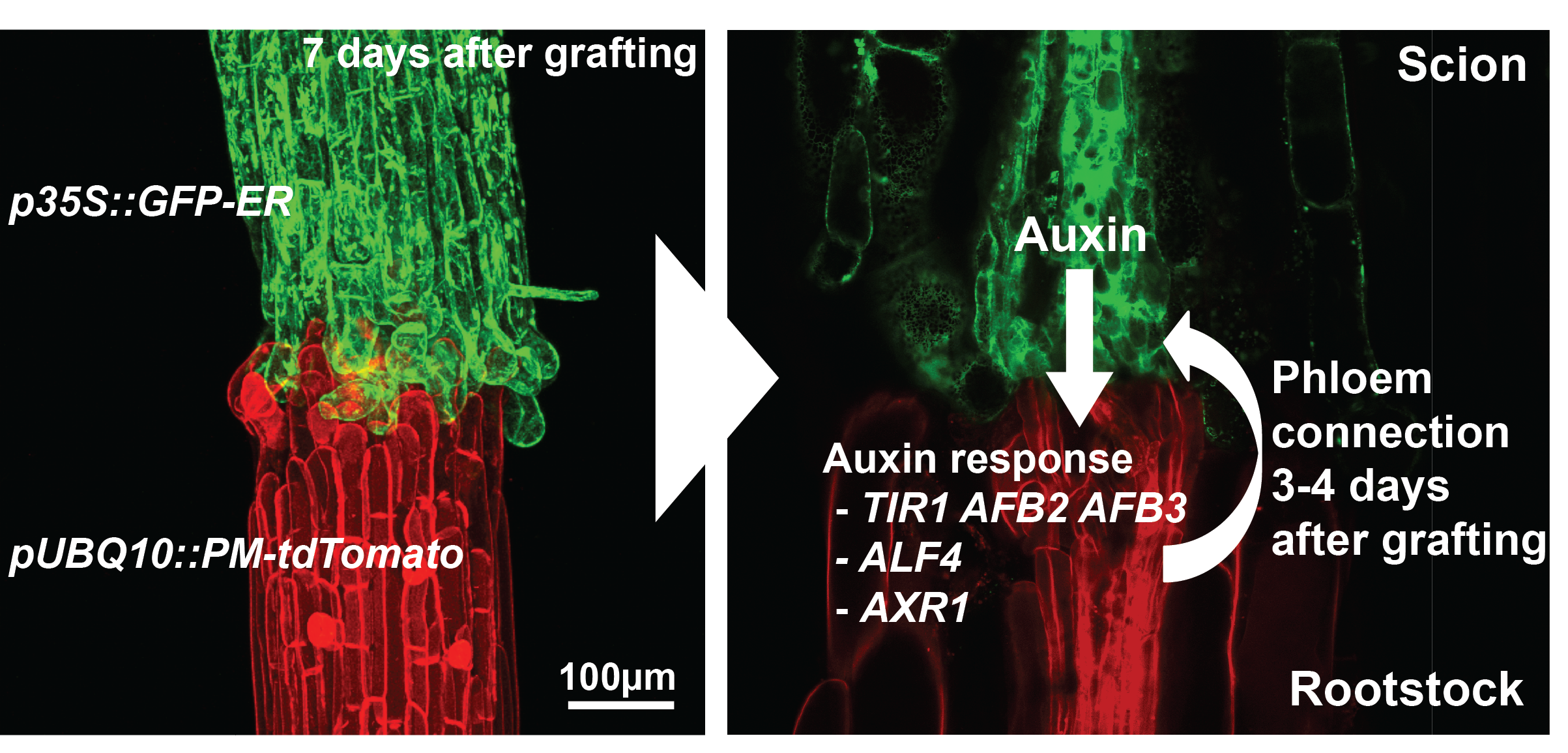
These results from our paper [10] described the dynamics of graft formation, but also established tools that we hope will be useful for the community to study graft formation. Our results also demonstrated how incredibly robust grafting is in young Arabidopsis hypocotyls: the majority of mutants, some of which are stunted and sick plants, grafted as robustly as wild type plants. The four genotypes out of 45 that affected phloem connection only delayed it by two-fold; none blocked the process. Lastly, it demonstrated an important role for auxin response in re-patterning vasculature and pointed to different mechanisms above and below the graft junction driving vascular connection.
The future for grafting
Grafting is a widely used technique in horticulture that is important for industries worth billions of dollars per year, including the wine and fruit industries. There are several ways research in grafting could benefit agriculture that should be future research priorities. Firstly, many graft combinations even within the same species are not successful and plant breeders have to develop new rootstocks that work well with a given scion. The molecular basis for this incompatibility and self/non-self recognition is unknown, but discovering the mechanism could reduce graft failure and allow wider grafts to be made between species in new combinations. For instance, wild relatives of our modern-day fruits and vegetables have often enhanced stress resistance that could be used as rootstocks to improve resistance in cultivated fruit trees, tomatoes, cucumbers, melons and potatoes. As grafting is widely conserved between dicots and gymnosperms (but interestingly not monocots), discoveries made in Arabidopsis might be conserved in other species. A future goal will be using tomato grafting as a platform to test discoveries made in Arabidopsis. In particular, understanding the mechanism and downstream targets of genes such as AXR1 and ALF4 may present targets to improve grafting efficiency.
Secondly, approximately 1% of flowering plants are parasitic [13]: they infect and attach to other species to draw out nutrients. Many parasitic plants form partial vascular connections including attaching their xylem to the host xylem [14], conceptually similar to graft formation. By better understanding how parasitic plants overcome the host/non-host recognition barriers, we could use this information to improve grafting. Similarly, blocking graft formation pathways may confer resistance to parasitic plants. Grafting is a fascinating biological process. By studying graft formation, we can better understand how plants heal wounds, repair vascular tissue, and distinguish self from non-self. Ultimately, basic research on grafting should improve and expand current grafting possibilities.
References
1. Mudge, K., Janick, J., Scofield, S., and Goldschmidt, E.E. (2009). A History of Grafting, Volume 35, (John Wiley & Sons, Inc.).
2. Wright, J.S. (1893). Cell Union in Herbaceous Grafting. Botanical Gazette 18, 285-293.
3. Moore, R. (1984). Ultrastructural Aspects of Graft Incompatibility between Pear and Quince Invitro. Ann Bot-London 53, 447-451.
4. Jeffree, C.E., and Yeoman, M.M. (1983). Development of Intercellular Connections between Opposing Cells in a Graft Union. New Phytol 93, 491-509.
5. Moore, R. (1984). A Model for Graft Compatibility-Incompatibility in Higher-Plants. Am J Bot 71, 752-758.
6. Kollmann, R., and Glockmann, C. (1985). Studies on Graft Unions .1. Plasmodesmata between Cells of Plants Belonging to Different Unrelated Taxa. Protoplasma 124, 224-235.
7. Pina, A., Errea, P., and Martens, H.J. (2012). Graft union formation and cell-to-cell communication via plasmodesmata in compatible and incompatible stem unions of Prunus spp. Sci Hortic-Amsterdam 143, 144-150.
8. Turnbull, C.G., Booker, J.P., and Leyser, H.M. (2002). Micrografting techniques for testing long-distance signalling in Arabidopsis. The Plant journal 32, 255-262.
9. Melnyk, C.W., and Meyerowitz, E.M. (2015). Plant grafting. Current biology : CB 25, R183-188.
10. Melnyk, C.W., Schuster, C., Leyser, O., and Meyerowitz, E.M. (2015). A Developmental Framework for Graft Formation and Vascular Reconnection in Arabidopsis thaliana. Current biology : CB 25, 1306-1318.
11. Nelson, B.K., Cai, X., and Nebenfuhr, A. (2007). A multicolored set of in vivo organelle markers for co-localization studies in Arabidopsis and other plants. The Plant journal 51, 1126-1136.
12. Segonzac, C., Nimchuk, Z.L., Beck, M., Tarr, P.T., Robatzek, S., Meyerowitz, E.M., and Zipfel, C. (2012). The shoot apical meristem regulatory peptide CLV3 does not activate innate immunity. Plant Cell 24, 3186-3192.
13. Westwood, J.H., Yoder, J.I., Timko, M.P., and dePamphilis, C.W. (2010). The evolution of parasitism in plants. Trends Plant Sci 15, 227-235.
14. Musselman, L.J. (1980). The Biology of Striga, Orobanche, and Other Root-Parasitic Weeds. Annual Review of Phytopathology 18, 463-489.