Understanding cell- and tissue-level decision-making – a tense and crowded situation in the skin!
Posted by Kate Miroshnikova, on 23 February 2018
The story behind our paper: Yekaterina A. Miroshnikova*, Huy Q. Le*, David Schneider*, Torsten Thalheim, Matthias Rübsam, Nadine Bremicker, Julien Polleux, Nadine Kamprad, Marco Tarantola, Irène Wang, Martial Balland, Carien M. Niessen, Joerg Galle & Sara A. Wickström. Adhesion forces and cortical tension couple cell proliferation and differentiation to drive epidermal stratification. Nature Cell Biology 20, p69–80 (2018)
Skin is a fascinating organ that dynamically adjusts its size throughout our lifetimes and does so perfectly while, at the same time, acting as a robust bi-directional barrier protecting us from the insults of the outside world. Of particular interest is the epidermis, which is the outermost, barrier- and load-bearing layer of the skin. Every time we bend our fingers, undergo cycles of weight loss or gain, or are a subject to life-altering events, such as pregnancy, the epidermis has to shrink, expand, and withstand various amounts of tension while still maintaining integrity! From a point of view of an engineer, skin is a marvel – how can this be? What is the structure of this stunning tissue allowing it to perform the many functions that it has?
The epidermis is a highly ordered, multi-layered stratified epithelium. The bottom-most sheet is a tightly packed layer of proliferating stem cells attached to both the underlying matrix as well as to the cells of the terminally differentiated, postmitotic suprabasal layer. Upon differentiation, cells delaminate from the basal stem cell layer and move upwards, filling the many layers of the skin. During this upward motility, the cells need to maintain tight cell-cell junctions in order not to compromise the barrier function of this epithelium. When reaching the most superficial layer, the cells lose their nuclei and dye, forming the dead cornified layer, from where the cells are slowly sloughed off. This turnover is quite rapid, and the entire epithelium is fully renewed every 7-10 days.
The basal layer is the hotspot of most of this activity in the epidermis where some stem cells divide and continue residing within the layer while others differentiate, delaminate, move upwards, and eventually die. In the homeostatic state, epidermis must maintain a constant tissue size and this means a relatively constant basal cell number. Intuitively, the rate of proliferation then must equal to the rate of departure and delamination of the differentiating cells. On a population-level this behavior is completely logical, but the decision-making occurs on cellular level. Then, what are the drivers of cellular fate decisions within a tissue and how do cells coordinate their cell fate with their position? This is the exactly the question that we tackled in our recent publication! It turned out that it is all about differential cell and tissue mechanics!
Let the force guide you – how to robustly couple position with fate
It is intuitively apparent why one may think about external forces, such as compression and tension, in the context of the basal layer of the epidermis as it is an incredibly crowded and mechanically-loaded tissue. It is also instinctive to consider differential cellular tension in the context of cellular extrusion as we now from ample developmental biology literature that cell sorting is largely based on differential cortical tension. To put it simply, it would be more likely for a soft cell to squeeze upwards from a tightly jammed space than it would be for a stiff one. Thus, we started off with the very basic hypothesis that differential cell mechanics would be an excellent cue guiding cells to either remain within the crowded layer or change their position and move upwards. This was especially compelling in the context of highly mitotic areas with highly elevated lateral cellular compression and cell shape deformation. Our hypothesis was that crowding could alter cell shape and cortical tension. Thus, the first question that we asked was whether there was mechanical difference between cells that start differentiating versus ones that remain stem cells within the basal layer. Using atomic force microscopy we observed a biphasic evolution of cortical tension of differentiating cells, where initially upon differentiation cells indeed became softer as they made the decision to detach from the layer and, upon successful escape from the basal layer, they became stiffer, while at the same time changing their adhesion profiles. By measuring adhesion forces we further realized that the more differentiated the cell, the stronger the cell-cell adhesion forces. This was exciting as it suggested that indeed cell fate changes were accompanied by changes in cell mechanics. It further suggested that perhaps the differentiated cell was not only being squeezed out of the stem cell layer, it could also be partially pulling itself out using the strong cell-cell adhesion forces.
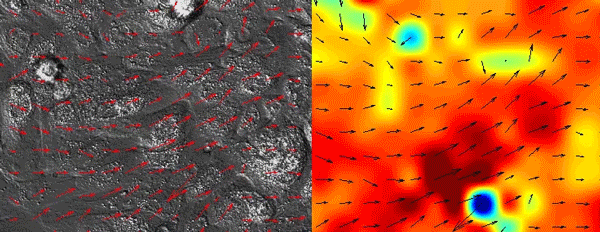
The path to finding answers: when physics meets cell biology
The next step was to ask the classical chicken-and-egg question of what comes first. Does the altered cell mechanics trigger differentiation or does differentiation trigger altered cell mechanics. To this end, we utilized a compression bioreactor as well as micro-patterned surfaces to precisely control crowding and cell shape anisotropy. The answers provided by these experiments made the engineers working on the project quite ecstatic as by artificially inducing crowding or restricting cell adhesion area and forcing cells to assume anisotropic shapes we were able to sufficiently to trigger differentiation. In addition, crowding was further, not surprisingly, sufficient to alter cell mechanics. So indeed, the stem cell layer crowding state seemed to control cell fate, and the cell fate change was coupled to a change in cell mechanics, providing an elegant mechanisms by which the tissue could monitor and react the changes in cell density as well as to couple cell fate to positioning.
As all of the initial observations were done using various in vitro manipulations, we subsequently turned to in vivo imaging of whole embryos to ensure that the in vitro principles are conserved and relevant to the state of the epidermis in vivo. Upon careful visual inspection of the in vivo epidermis, it was immediately apparent that the basal layer existed in a solid-like, jammed state. Interestingly, we subsequently noted that jamming coincided with the onset of delamination in our in vitro monolayers, implying that delamination of differentiating cells could be a specific feature of jammed epithelia. The biological effects of these imaging experiments were clear but their quantification was a bit more of a challenge that we overcame via a fruitful collaboration with Dr. Irene Wang and Dr. Martial Balland from Grenoble, France that allowed us to model tissue strains and monolayer flows in a quantitative manner.
Finally, it was important to test whether crowding-induced stochastic decrease of cortical tension coupled to increased cell-cell adhesion was sufficient to induce delamination. This was challenging, as any chemical or genetic perturbation would affect the mechanics of all cells, whereas we needed to induce mechanical heterogeneity. So to get an initial hint that we were really on to something relevant, we initiated an collaboration with Dr. Joerg Galle and Dr. Torsten Thalheim from Leipzig, Germany who had in the past beautifully simulated intestinal stem cell homeostasis in silico. They set out to simulate monolayer crowding, where increasing cell density through proliferation would, as shown by our experiments, reduce cell surface area, reduce cortical tension and increase cell-cell adhesion.
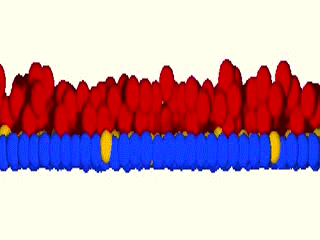
As a matter of fact, we were blown away by the results that the system maintained epidermal homeostasis at rates comparable to what had previously been observed using live imaging of intact epidermis of mice, and was completely buffered against changes in proliferation rates within the basal layer. Further, only cells with low cortical tension and high cell-cell adhesion delaminated, showing that this could indeed be an extremely robust mechanism to couple cell fate with position. We further went on to validate key aspects of the model by cell mixing experiments in epidermal organotypic cultures, where we observed that single E-cadherin-deficient cells, that showed lower cell-cell adhesion forces and high cortical tension, had severely compromised ability to delaminate, even if they had terminally differentiated.
Taken together, we conclude that individual cells tune their fates based on the activities of the neighboring cells, and by changing their mechanical properties as part of this fate switch, change their position within the tissue. This is quite remarkable, and we are now thinking about the implications of this phenomenon in terms of tissue pathologies. For example, recent findings by the lab of Dr. Phil Jones demonstrated that sun-exposed skin of healthy individuals contains clonal patches of cells with cancer-causing mutations such as mutations in Ras, but these clones co-exist with normal cells without any perturbations to the physiological functions or structure of the epidermis. Our skin is seems thus to be buffered against perturbations such as local differences in proliferative activity or differentiation rates.
I am grateful for having had the chance to work together in a fantastic team of engineers, physicists, biochemists, and cell biologists and am particularly proud to have been leading a study that employed such a wide spectrum of techniques from in vivo imaging of whole organisms to developing new quantitative algorithms and agent-based simulations to explore and understand the wonders of biology.