It’s in the head: How male and female fruit flies grow apart
Posted by Annick Sawala, on 7 December 2017
A discussion of our recent paper: Annick Sawala & Alex P. Gould (2017). The sex of specific neurons controls female body growth in Drosophila. PLoS Biology, October 4 2017.
In the beginning…
The story behind this study provides yet another example of where the pursuit of a few chance observations developed into an interesting project in its own right.
I started my postdoc in the lab of Alex Gould at The Francis Crick Institute with the objective of investigating how nutrient deprivation affects the growth of different body parts of the fruit fly larva. These experiments required precise measurements of organ sizes, and it soon became pretty clear that in order to get the most useful data, males and females had to be analysed separately. Not because the different sexes necessarily show different growth responses to starvation, but because female body parts are consistently 20-40% larger than their male counterparts. Hence, if males and females are pooled, the data have a large variance. Surprisingly, a lot of studies that have analysed larval growth seem to have ignored this sex difference in body size, officially termed sexual size dimorphism (SSD). So SSD effectively doubled my workload but it also opened up a new question: how does the sex of the larva influence its growth?
Summary of the key findings
We used two opposite angles of attack to identify the mechanism underlying larval SSD. First, in a sort of top-down approach, we asked if there were any measurable differences in growth parameters or behaviour that could give insights into underlying genetic mechanisms. Second, using a bottom-up strategy, we manipulated sex determination genes in individual cell types to see if the sex of a specific body part influenced overall body size.
For the first approach, I painstakingly weighed individual male and female larvae at different stages in order to determine their growth curves. When a larva hatches from the embryo it only weighs around 10mg, about 1/5th of the mass of a grain of salt! [1], and it grows to a final size of about ~2mg, around half the mass of a sesame seed [2], before it undergoes pupal development about 4 days later. This work established that males and females have the same size at larval hatching and that they have similar time windows of growth. Nevertheless, females begin to have a higher growth rate from about the middle of the second larval instar onwards. Interestingly, I found that the greatest difference in the fold rate of growth between the sexes occurs relatively early in larval development, during the second larval instar. This means that any pathways that control sex differences in body size should be active before or during the second larval instar.
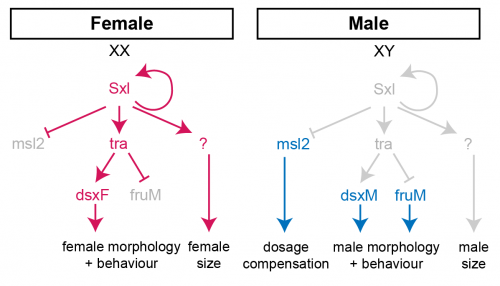
In females, the presence of two X-chromosome activates the expression of the splicing factor Sex-lethal (Sxl). Sxl maintains its own expression and causes sex-specific splicing of transformer (tra), such that a functional Tra protein is only produced in females. Tra is another splicing factor that causes the sex-specific splicing of the transcription factors doublesex (dsx) and fruitless (fru), which regulate sex-specific morphologies and behaviour. Sxl also represses the translation of male-specific lethal 2 (msl-2) in females, thereby limiting assembly of the X-chromosome dosage compensation complex to males.
For the second approach, we used the amazing GAL4/UAS system of Brand and Perrimon [3] to manipulate a gene called Sex-lethal (Sxl), the master regulator of fly sex determination. Sxl is normally only expressed in females, and it directs the sex-specific splicing of downstream genes in order to specify the development of female morphology and behaviour (Fig 1). Sxl mutant females are masculinised both in terms of their morphology and their body size, demonstrating that Sxl controls SSD by promoting higher female growth.
I used RNAi to inhibit Sxl expression in different tissues and, strikingly, found that knockdown with a pan-neuronal Gal4 driver reduced the body size of females to that of males (Fig 2A). In the reverse experiment, restoring Sxl expression in neurons could partially rescue the female body size of Sxl mutants. Thus, Sxl acts in neurons in a non-cell autonomous manner to boost the growth of the female body. To pinpoint the neuronal population in which Sxl functions to promote SSD, a panel of neuronal subset-specific Gal4 drivers were then screened. We found that Sxl acts additively in at least two non-overlapping subsets of neurons: 1) the insulin producing cells (IPCs), a cluster of 7 neurosecretory cells that are known to secrete several insulin-like peptides into the circulation, and 2) cells expressing the Gad1-Gal4 driver, which is active in GABAergic neurons (although we found that the overlap with GABA-expressing neurons is only partial).
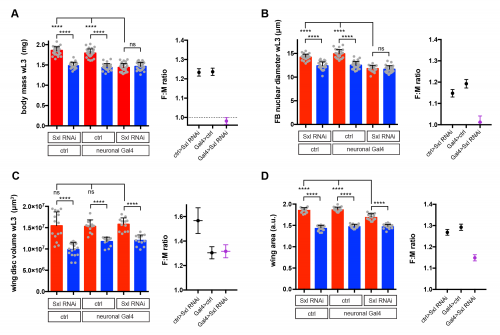
Sxl RNAi was expressed using a pan-neuronal Gal4 driver (elav[c155]-Gal4) and the effect on SSD was measured for various body parts. Measurements were taken at the end of larval development, in wandering L3 (wL3) larvae (A-C) or in adult flies (D). The right graphs in each panel plot the female to male ratio as a quantification of SSD. Pan-neuronal Sxl RNAi abolishes SSD at the level of larval body mass (A) and the fat body nuclear diameter (B), while SSD of the wing imaginal disc remains intact (C). Pan-neuronal Sxl RNAi reduces, but does not abolish SSD of the adult wing (D).
The results of both approaches raise four tricky questions that Alex and I have been thinking about, and also investigating, with varying levels of success:
How does the new study fit with the dogma that somatic sex determination in flies is cell-autonomous?
We think the answer to this important question lies in differences between how Sxl regulates the SSD of the larval versus the adult body. On the face of it, our finding that Sxl acts in neurons to increase female body growth would seem to conflict with classic genetic studies of gynandromorphs (flies mosaic for male and female cells). These sex mosaic flies had clearly demonstrated that male/female morphologies are controlled cell-autonomously. Not only that but, in a bilateral gynandromorph, the male side is smaller than the female side [4] (Fig 3). So how can we square the classic findings with our new observations?
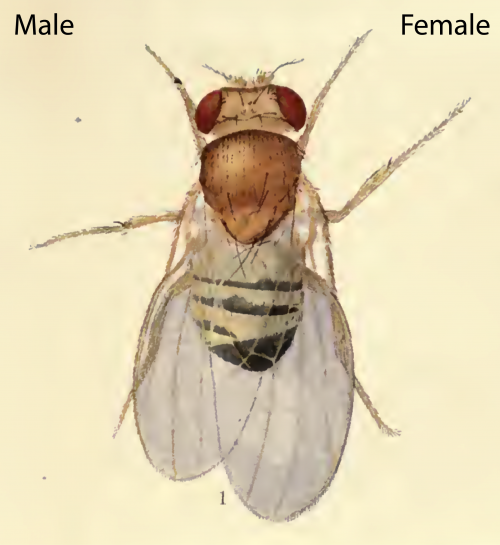
The drawing depicts a mosaic fly with male cells developing on the left side and female cells developing on the right side, likely as a result of a loss of one X-chromosome during early embryonic divisions. Note that the male side shows typical male morphological differentiation such as the sex-combs (dark bristled on front leg), as well as a smaller body size compared to the female side.
The first thing to note is that the presence of the cell-autonomous effects revealed by gynandromorphs does not completely rule out additional non-cell autonomous effects. In fact, such a dual SSD mechanism does seem to operate in mammals. For example, a recent mouse study suggests that gonadal hormones and direct effects of sex chromosomes combine to produce overall differences in growth and metabolism between males and females [5, 6]. Furthermore, we and others [7, 8] have found that manipulation of Sxl or its downstream target transformer does indeed have cell-autonomous effects on growth, although they are too small to account for the full extent of SSD.
Secondly, we realised that the gynandromorph studies only looked at the external structures of the adult fly. These are derived from small groups of diploid cells called imaginal discs, which grow within the larval body and only transform into adult structures during metamorphosis. In contrast, I had measured SSD for the larval body itself, the bulk of which is composed of large polyploid cells, which are degraded during metamorphosis and so do not make it to the final fly. This realization led to the testable hypothesis that SSD is regulated differently in the larval polyploid tissues versus the diploid imaginal tissues. Unlike many of our crazy hypotheses, this one turned out to stand the test of time as neuronal knockdown of Sxl abolished SSD in the fat body (a larval tissue), yet it had no effect on SSD in the wing imaginal disc (the adult wing precursor) (Fig 2B and 2C). Thus, neuronal Sxl in females specifically boosts the growth of larval not imaginal tissues during the period of juvenile development. Nevertheless, by the time the imaginal discs have transformed into the mature external structures of the adult fly, I did observe a moderate effect of neuronal Sxl knockdown on SSD (Fig 2D). To account for this, we propose that, by promoting larval tissue growth, neuronal Sxl also indirectly boosts the growth of imaginal tissues, because a larger larval body confers increased pupal resources (nutrients and/or growth factors) to sustain the growth of imaginal structures during pupal stages. An alternative explanation, which we cannot rule out for now, is that neuronal Sxl also acts during pupal stages to directly influence the size of imaginal structures.
Is there a role for insulin signalling in SSD?
We think the overall answer to this question is “yes but only for imaginal tissues” and here’s our logic for why this may be the case. Sxl functions in insulin producing cells and it is known that insulin secretion from these cells is a key regulator for growth, so an attractive hypothesis is that Sxl promotes insulin secretion in females, thereby boosting female growth. Interestingly, SSD in mammals is driven in part by gonadal hormones causing sex differences in insulin-like growth factor 1 (IGF-1) release. However, our data suggest that SSD of the larval body (i.e. the target of neuronal Sxl) is established independently of sex differences in insulin signalling. This is because, at the early stages when the sex difference in growth rates is maximal (the second instar larva), no sex differences in insulin secretion or insulin signalling could be detected. In addition, several manipulations aimed at changing insulin secretion from IPCs also had no effect on larval body SSD. And finally, mutant larvae that lack one or all of the insulin-like peptides (Ilps) produced in the insulin producing cells are very small yet their SSD remains intact. These findings suggest to us that Ilps are not required for the establishment of sex differences in growth in the early larva.
But the twist here is that there is evidence suggesting a role for insulin signalling in SSD in older larvae and in adult flies. Thus, insulin receptor mutant adult flies show a strong reduction in SSD (measured as dry body mass) [9]. At late larval stages, it has also been reported that insulin secretion from IPCs and insulin signalling is higher in females [7]. It is therefore likely that sex differences in insulin signalling in old larvae contribute to SSD in imaginal tissues, even though at this time larval body growth has largely finished. In support of this, I found that when IPCs are genetically manipulated to decrease their size and so presumably their insulin secretion, SSD is abolished in the wing imaginal disc but it remains intact in the larval body, despite the smaller absolute body masses of both sexes. Clearly, more work is needed to understand why insulin signalling appears to regulate the SSD of larval versus imaginal tissues so differently.
What is downstream of neuronal Sxl to boost female body growth?
We wish we could answer this tricky question but, unfortunately, all we can give you right now is a list of factors that are probably NOT involved.
As mentioned above, the current evidence indicates that insulin like peptides are not strong candidates for the Sxl targets in IPCs that are relevant for establishing larval body SSD. Nevertheless, it is possible that Sxl regulates the secretion of other signalling molecule(s) into the circulation, either directly from IPCs, or from cells that the IPC neurons connect with. Notably, IPCs send axons to the ring gland [10, 11], a major hemosecretory organ, which could provide such a systemic signal. The role of Sxl in the Gad1-Gal4 neurons is even less clear.
Two direct downstream targets of Sxl that have been widely studied are transformer and msl-2 (see Fig 1). Our loss of function and rescue experiments strongly suggest that neither transformer nor msl-2 play a role in the neurons regulating larval SSD. The msl-2 data also ruled out the possibility that the reduction in female body sizes we saw in Sxl loss of function experiments were due to “sickness” caused by ectopic activation of msl-2 expression and the dosage compensation machinery. It is likely that a new Sxl target is involved, but identification of this may well require unbiased sequencing experiments or other genome-wide techniques, using brains from the relevant developmental stage.
Is increased female body growth driven by increased feeding?
We have been asked this question a lot at conferences. We did measure feeding rates in early second and early third instar larvae. In both cases, sex differences in the absolute food intake rate (i.e. intake per larva) were detected, but when these were normalised for body mass, mass-specific food intake rates (i.e intake per mg of larval tissue) appear to be the same for males and females. This suggests to us that sex differences in absolute food intake are a consequence of a larger female body mass, but not the driver of it. So why then do female larvae have a higher mass-specific (i.e. fold) growth rate than males despite both sexes having similar mass-specific feeding rates? One possible explanation here is that female larvae are more efficient at converting nutrients into tissue mass than males.
It is important to highlight that there are caveats to our conclusion that mass-specific food intake is equal in male versus female larvae. This is because we measured food intake over 20-30 minutes using a blue dye uptake method that would not be sensitive enough to detect very small differences. Some clever method for measuring food consumption over much longer periods might be necessary to detect very small sex differences in mass-specific food intake. Unfortunately, this may be tricky as larvae not only eat their food but they also burrow in it, and defecate into it. However, there is another reason for thinking that the neuronal Sxl mechanism for establishing SSD does not involve sex-specific feeding. This is because increased feeding rates in females would be predicted to increase the growth of both larval and imaginal tissues, yet we observed that imaginal tissues inside the larva are blind to neuronal Sxl regulation.
Concluding remarks
Sex determination and growth regulation have been extensively studied in Drosophila for many years. It is therefore very surprising that only a handful of studies have investigated how sex influences growth in this model organism. The neuronal Sxl relay mechanism that we have recently discovered contrasts with the text book view that somatic sexual differentiation in insects is controlled in a strictly cell-autonomous fashion. Future research into the neuronal Sxl mechanism for larval SSD will be needed to identify the relevant Sxl targets and perhaps endocrine growth regulatory mechanisms that act selectively on larval polyploid tissues rather than diploid imaginal discs. Despite this, our research has already revealed glimpses that suggest that the regulatory logic of SSD in mammals and insects may be more similar than previously thought. This encouraging news opens up the possibility of using our favourite model organism to study how sex differences in growth and metabolism impact upon disease – an important emerging area of biomedical research.
References
- “Grain of salt”: Bluebulb Projects’ The Measure of Things; [02.12.2017]. Available here.
- “Sesame seed” [02.12.2017]. Available here.
- Brand AH, Perrimon N. Targeted gene expression as a means of altering cell fates and generating dominant phenotypes. Development. 1993;118(2):401-15.
- Morgan THB, C.B. I. The Origin of Gynandromorphs. Contributions to the genetics of Drosophila melanogaster. 278: Publs Carnegie Instn; 1912. p. 1-122.
- Chen X, McClusky R, Chen J, Beaven SW, Tontonoz P, Arnold AP, et al. The number of x chromosomes causes sex differences in adiposity in mice. PLoS Genet. 2012;8(5):e1002709. doi: 10.1371/journal.pgen.1002709.
- Arnold AP, Chen X, Link JC, Itoh Y, Reue K. Cell-autonomous sex determination outside of the gonad. Dev Dyn. 2013;242(4):371-9. doi: 10.1002/dvdy.23936.
- Rideout EJ, Narsaiya MS, Grewal SS. The Sex Determination Gene transformer Regulates Male-Female Differences in Drosophila Body Size. PLoS Genet. 2015;11(12):e1005683. doi: 10.1371/journal.pgen.1005683.
- Regan JC, Khericha M, Dobson AJ, Bolukbasi E, Rattanavirotkul N, Partridge L. Sex difference in pathology of the ageing gut mediates the greater response of female lifespan to dietary restriction. Elife. 2016;5:e10956. doi: 10.7554/eLife.10956.
- Testa ND, Ghosh SM, Shingleton AW. Sex-Specific Weight Loss Mediates Sexual Size Dimorphism in Drosophila melanogaster. PLoS One. 2013;8(3):e58936-e. doi: 10.1371/journal.pone.0058936.
- Rulifson EJ, Kim SK, Nusse R. Ablation of insulin-producing neurons in flies: growth and diabetic phenotypes. Science. 2002;296(5570):1118-20. doi: 10.1126/science.1070058.
- Cao J, Ni JL, Ma WX, Shiu V, Milla LA, Park S, et al. Insight into Insulin Secretion from Transcriptome and Genetic Analysis of Insulin-Producing Cells of Drosophila. Genetics. 2014;197(1):175-92. doi: 10.1534/genetics.113.160663.