A Transgenic Optical Tool Planning Resource
Posted by Kelli Fenelon, on 3 July 2024
Since the sequencing of GFP in the 1990’s, interrogation of biological questions using transgenic model organisms expressing genetically engineered fluorescent molecules has exploded across many biological fields. Living organisms are superiorly suited to these inquiries as they’re the native environment of the mysteries being explored. A myriad of biological tools, designed to elucidate these perplexities of nature, have been developed, but most exciting amongst them are the burgeoning systems of optical tools for use in live organismal studies. To address the necessity for a chronicled repertoire of the tools in this toolkit, in a recent review, KD Fenelon et al. 2024 compiled a reference for scientists seeking to understand or develop new transgenic models expressing optical tools for visualization, quantification, and/or manipulation of subcellular and tissue-level molecular processes in vivo 1. These purely transgenic tools can be generally broken up into three categories: tags for visualizing biological phenomena, sensors for measuring biological function, and optogenetics for manipulating biological processes (Fig. 1).
Tagging systems have advanced dramatically from the days of basic fluorescent protein fusions to label the fused protein. The slow maturation rates of fluorescent proteins has been remedied through development of secondary attachment fluorescent systems whereby an aqueous pool of fluorescent proteins is maintained which produces sharp puncti through binding to target proteins. Additionally, ever more creative manipulations and modifications of the Cas9 enzyme allows for easy and efficient labeling of DNA sequences. Furthermore, transcription and RNA dynamics can be visualized in vivo through the use of several available tagging techniques, including by introducing stem loops to an RNA sequence to be bound by a fluorescently labeled coat protein or by engineering the RNA binding domain of the Pumilio system to label endogenous sequences.
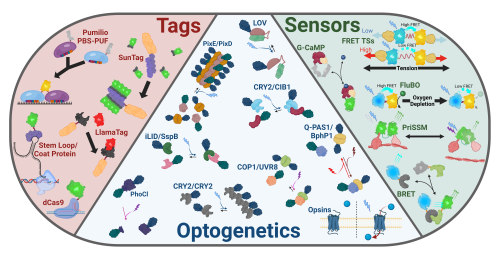
Indeed, fluorescent labels have further evolved to be utilized as sensors to measure molecular-level phenomena2. Forster Resonance Energy Transfer (FRET) and Bioluminescence Resonance Energy Transfer (BRET) allow for measurement of distances between compatible fluorescent and bioluminescent proteins which are used to sense a myriad of physical phenomena including proximity, tension, and metabolite levels. Furthermore, split and circularly permuted fluorophore allow for proximity and conformational detection.
Perhaps most excitingly, optically sensitive proteins have now been leveraged to facilitate light-mediated, physical control of fusion protein constructs. These extraordinary tools are broadly labeled ‘optogenetics’ and enable a wide-range of external subcellular manipulations3. Optogenetic systems, such as PixD, CRY2/CIB1, iLID/SspB, COP-1/UVR8, & Q-PAS1/BphP1, enable light-dependent, reversible binding/dissociation of fusion protein constructs. Similarly, PhoCl facilitates irreversible, light-dependent cleavage of fusion protein constructs. Analogous to these, systems such as PixE/PixD and CRY2 facilitate light-dependent oligomerization of proteins. Optogenetic systems including the light-oxygen-voltage (LOV) domain family sterically rearrange to ‘hide’ or expose protein sequences within a cryptic domain in response to light. Further, a wide variety of naturally occurring and genetically engineered opsins can now be used to induce membrane transfer of a wide variety of ions and metabolites via light exposure.
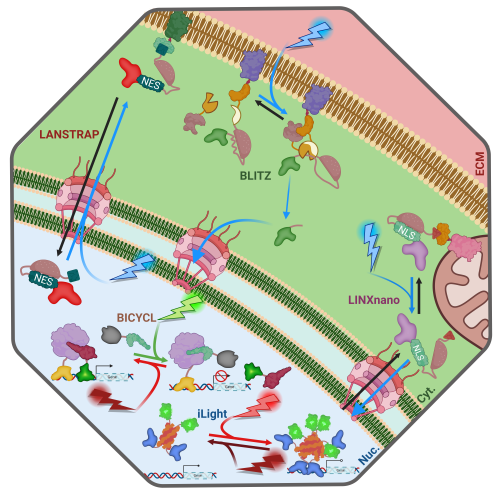
Already, there is an explosion of new and exciting innovative and complex applications for these tools (See Fig. 2). For example, LANSTRAP4 facilitates light-dependent nuclear export and attachment to the cell membrane of transcription factors while LINXnano5 facilitates light-induced mitochondrial membrane detachment and nuclear import of target transcription factors. Another inventive example is the BLITz6 system which keeps proteins tethered to the cell membrane until light exposure induces an irreversible cleavage via a split protease. Further, gene expression can be controlled without requiring cytoplasmic accumulation of transcription factors: BICYCL7 and iLight8 facilitate toggling gene expression on an off by changing the wavelength of light exposure. These are but a few of the exciting and invaluable tools currently available to the modern genetic engineer, warranting their compilation in the resource published recently by the Koromila Lab9,10 in PLOS Genetics.
References
- Fenelon, K.D., Krause, J. & Koromila, T. PLoS Genet 20, e1011208 (2024).
- Wang, M., Da, Y. & Tian, Y. Chem Soc Rev 52, 1189–1214 (2023).
- Fischer, A.A.M., et al.. Curr Opin Chem Biol 70, (2022).
- Yumerefendi, H. et al. ChemBioChem (2018).
- Yumerefendi, H. et al. Nat Chem Biol 12, (2016).
- Lee, D. et al. Nat Methods 14, 495–503 (2017).
- Jang, J. et al. Nat Methods 20, 432–441 (2023).
- Kaberniuk, A.A.,et al. Nat Commun 12, 1–12 (2021).
- Fenelon, K.D. et al. Biol Open 11, (2022).
- Stevens, L.M. et al. PLoS Genet 17, e1009544 (2021).
Biorender and Adobe Illustrator were used in creation of the illustrations.
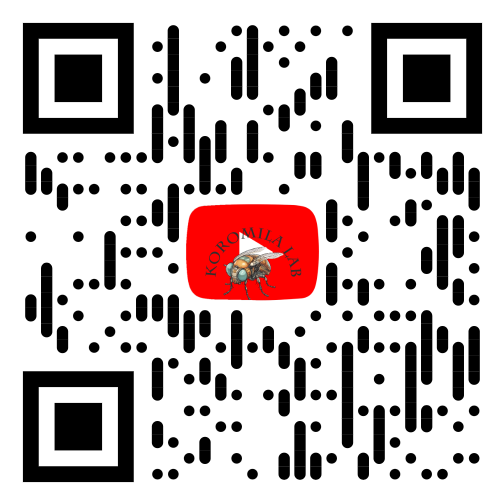