A day in the life of a Capitella teleta lab
Posted by alexislanza, on 10 July 2018
It’s undoubtedly the middle of summer here in Saint Augustine, Florida. Daily temperatures are soaring into the 90s, and we’re grateful if the humidity dips below 70%. Thankfully, the Seaver lab doesn’t have to contend with much of this heat. Instead, our members are inside, comfortable though busier than ever, mentoring summer interns, piloting new experiments, and writing up papers. It’s summertime, and the University of Florida’s Whitney Laboratory for Marine Bioscience is bustling with research as a whole!
I am Alexis Lanza, a PhD candidate in Dr. Elaine Seaver’s Lab with a research interest in embryonic signaling events critical for dorsal ventral axis formation in annelids. Here, I share a bit about our lab and its daily activities.
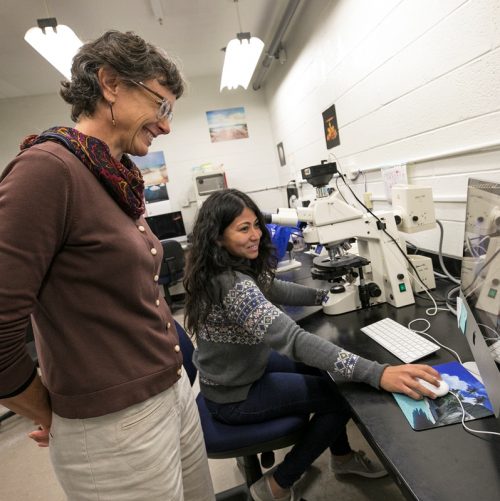
Area of Research
Broadly speaking, the research being done in the Seaver lab aims at answering questions about the development and evolution of marine invertebrates. We’re curious about the cellular and molecular mechanisms that drive developmental processes from an unfertilized egg through to zygotic, larval, and juvenile stages, as well as during regeneration. We’re also interested in how molecular differences that exist across species may account for body plan differences across animals. In our lab we use a small marine worm called Capitella teleta as our model organism.
Capitella teleta as an ideal model
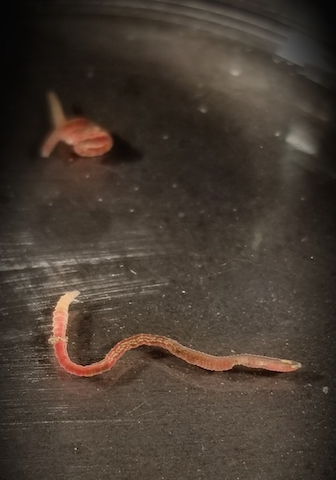
Capitella teleta is a polychaete annelid worm and member of the
Lophotrochozoa. It is a cosmopolitan species and can be found along intertidal zones living in organically rich marine sediments. Capitella can easily be kept as part of a lab colony in glass finger bowls with seawater and roughly a tablespoon of mud. Following fertilization, its embryos develop into pelagic non-feeding larvae that metamorphose into juvenile worms approximately 9 days post-fertilization. At around 8 weeks old, these juveniles mature into adults that are easily distinguishable by sex and are capable of reproducing year-round. Capitella’s ability to produce embryos regularly is one reason why it is an ideal candidate for early developmental studies.
Furthermore, its embryos undergo a stereotypic cleavage program called unequal spiral cleavage in which blastomere formation occurs according to a predictable order, size, and position. This predictable cleavage program is also shared by other taxa including mollusks and nemerteans, and allows for the identification of individual blastomeres that can then be microinjected, or deleted using a laser (Meyer and Seaver, 2010; Yamaguchi et al., 2016).
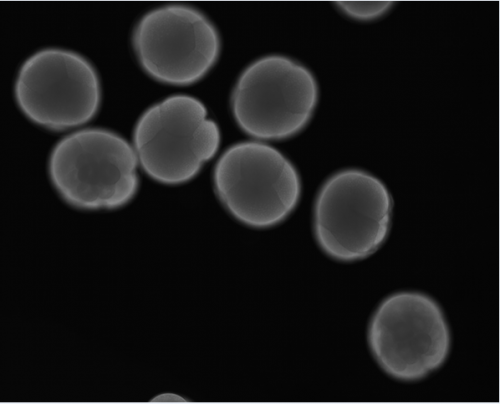
Another prominent area of study within our lab focuses on how regeneration occurs in Capitella (de Jong and Seaver, 2017). Capitella, like many of its annelid counterparts, possesses regenerative capabilities following transverse amputations, as it is capable of regenerating posterior segments. However, Capitella cannot regenerate anteriorly. The development of Capitella as an annelid model for developmental and regeneration studies was rooted most significantly in our ability to conduct experimental manipulations, functional genomics, as well as having a sequenced, annotated, and slowly evolving genome (Simakov et al., 2012). Altogether, the Seaver lab utilizes tools of experimental embryology, molecular and cellular biology, and functional genomics to answer evo/devo questions.
A typical day of animal care
Maintaining a Capitella colony requires several activities including feeding. While these tasks aren’t difficult in themselves, every member of the Seaver lab chips in throughout the week, tackling a different task during breaks in experiments.
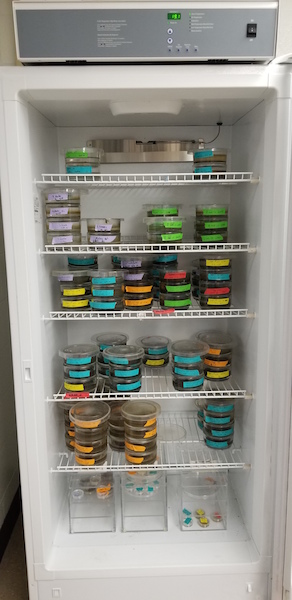
Sifting
Every glass finger bowl initially contains 40 individual worms. As the worms become reproductive however, we need to make an effort to avoid overpopulation in each bowl. When “sifting,” worms are removed from their bowl of mud and carefully sorted under a dissecting microscope. Unhealthy worms and larvae (the product of spontaneous matings) are removed, and only healthy individuals are placed into a fresh bowl of mud to be restocked as part of the working colony used for experiments.
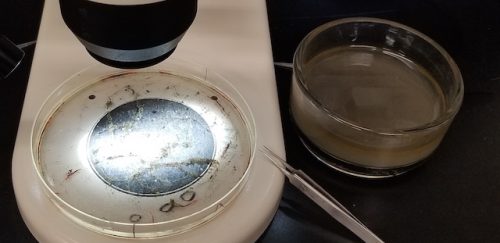
The Seaver lab divides its colony of bowls into two groups, each of which is sifted on an alternating, bi-weekly schedule. At the start of each week, a table listing all the colony bowls that need to be sifted that week is made.
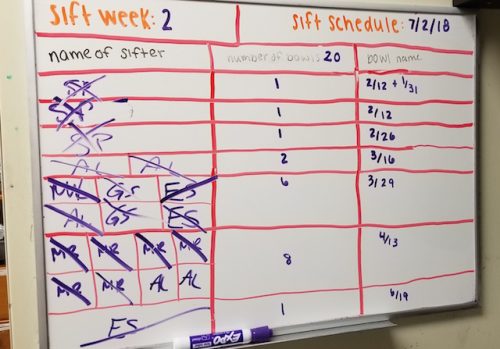
Lab members will then sign up for particular bowls based on their experimental needs are for the week. For instance, colony bowls with worms that have only recently reached sexual maturity are less likely to have larvae than would older bowls. Thus, if aiming to collect larvae one might want to sift an “older bowl.” Alternatively, if the goal is to conduct embryological experiments one may want to collect mature male and female worms, which can be used as a mating pair to produce early stage embryos.
Setting up the next generation
To maintain our lab colony through generations, it’s crucial that we plan ahead. As our worms age, they become less healthy and produce less offspring so are gradually phased out via the sifting process. To account for losing these individuals we introduce young, sexually mature worms (approx. 8 weeks old), all of which were raised from larval stages.
During the sifting process, lab members collect late stage larvae (approx. 8-9 days old), which are then used to create the new generation of colony bowls. First, glass fingerbowls are filled with seawater plus a teaspoon sized scoop of mud. Next, late stage larvae from several different mating pairs are pipetted into said fingerbowls until the total number of larvae equals 40. The mud is used to induce larvae to metamorphose. These new bowls will then be raised at 16°C for 8 weeks before being incorporated into the working colony.
Feeding
Animals need to be fed fresh mud once a week. We collect, sieve and freeze large quantities of marine mud that can then be thawed and used for animal care over the course of several months. To feed the colony, seawater is first decanted from each bowl. One tablespoon of mud is then added to each bowl, followed by the addition of fresh seawater. This process takes anywhere from 10 minutes to 1 hour depending on how large the colony is at any given time.
Fingers crossed for embryos
Mating Bowls
Remember those mature male and female worms collected during the sifting process? To acquire early cleavage stage embryos, mature male and female worms are separated by sex into bowls with 4 or 5 individuals each for about 3 days. When ready to set up a mating we simply combine the individuals from a male bowl with those from a female bowl. In general, we’ve noticed that it takes about 8-10 hours for the worms to mate and the females to lay their broods. Since most of our embryology experiments begin in the morning we need to set up these matings around midnight… this often means taking your worms home with you and only giving vague answers to questions your housemates may ask.
In the morning, back at the lab, we sift our mating bowls with bated breath to check if any of the female worms laid a brood of fertilized embryos.
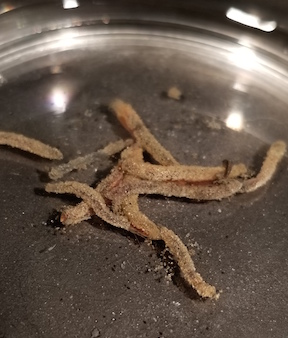
Using our dissecting microscopes and a pair of forceps, we dissect open any brood tubes and gently pipette the embryos into a clean petri dish. From here we can stage the embryos and determine how many cleavage divisions into development they are. Capitella embryos are approximately 200 microns in size and each cleavage division takes approximately 45 minutes. These embryos are amenable to chemical perturbants, allowing us to manipulate various signaling pathways and determine its role in development (Lanza and Seaver, 2018). Furthermore, Capitella embryos can be microinjected. To do so, the egg membrane of the embryo is first softened using a sucrose: sodium citrate mixture. This treatment is typically performed one cleavage division before the desired cell stage you wish to inject. We can then microinject individual cells during early cleavage stages with aqueous or lipophilic lineage tracers, DNA, RNA, or morpholinos, and then raise these embryos in seawater to larval stages (Meyer et al., 2010; Seaver, 2016). Like in many other species, most of the difficulty about working with early cleavage stage embryos is timing related. Since we can’t predict exactly when the eggs are fertilized we often end up racing against the clock to get our experiments underway before our target cleavage cycle ends or wait for hours until our embryos finally make it to the target cell stage. In general, after experimental manipulation, embryos are raised for six days to larval stage before being fixed for phenotypic analysis.
By the day’s end, the afternoon showers have usually subsided and the temperature has waned. It’s around then that the members of the Seaver lab emerge – just in time to enjoy the last few hours of sunlight during another Florida summer.
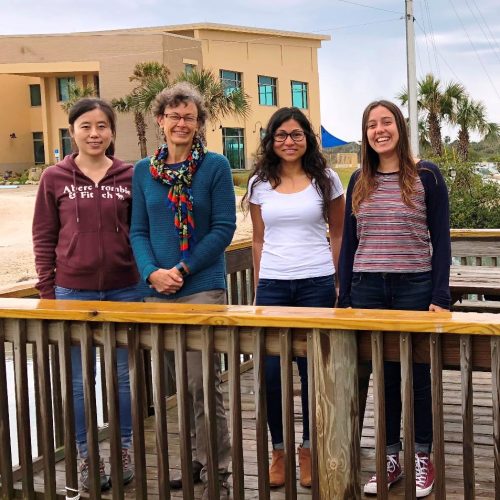
References
de Jong, D.M., Seaver, E.C., 2017. Investigation into the cellular origins of posterior regeneration in the annelid Capitella teleta. Regeneration 61–77. doi:10.1002/reg2.94
Lanza, A.R., Seaver, E.C., 2018. An organizing role for the TGF-β signaling pathway in axes formation of the annelid Capitella teleta. Dev. Biol. 1–15. doi:10.1016/j.ydbio.2018.01.004
Meyer, N.P., Boyle, M.J., Martindale, M.Q., Seaver, E.C., 2010. A comprehensive fate map by intracellular injection of identified blastomeres in the marine polychaete Capitella teleta. Evodevo 1, 8. doi:10.1186/2041-9139-1-8
Meyer, N.P., Seaver, E.C., 2010. Cell lineage and fate map of the primary somatoblast of the polychaete annelid Capitella teleta. Integr. Comp. Biol. 50, 756–767. doi:10.1093/icb/icq120
Seaver, E.C., 2016. Annelid models I : Capitella teleta. Curr. Opin. Genet. Dev. 39, 35–41. doi:10.1016/j.gde.2016.05.025
Simakov, O., Marletaz, F., Cho, S.-J., Edsinger-Gonzales, E., Havlak, P., Hellsten, U., Kuo, D.-H., Larsson, T., Lv, J., Arendt, D., Savage, R., Osoegawa, K., de Jong, P., Grimwood, J., Chapman, J.A., Shapiro, H., Aerts, A., Otillar, R.P., Terry, A.Y., Boore, J.L., Grigoriev, I. V., Lindberg, D.R., Seaver, E.C., Weisblat, D.A., Putnam, N.H., Rokhsar, D.S., 2012. Insights into bilaterian evolution from three spiralian genomes. Nature 493, 526–531. doi:10.1038/nature11696
Yamaguchi, E., Dannenberg, L.C., Amiel, A.R., Seaver, E.C., 2016. Regulative capacity for eye formation by first quartet micromeres of the polychaete Capitella teleta. Dev. Biol. 410, 119–130. doi:10.1016/j.ydbio.2015.12.009