Conflict between neighbours: a perfect way of rebuilding a complete organ system
Posted by the Node, on 16 November 2022
In their recent manuscript, published in Developmental Cell, Vijina Varapparambath, Mabel Maria Mathew, Anju Pallipurath Shanmukhan, and colleagues explore the mechanism underlying de novo shoot regeneration. They discover that mechanical feedback between two populations of juxtaposed cells – one which will eventually become the shoot and the other its neighbors – is what propels fate changes and sculpts the regenerating shoot meristem. Now, one of the co-first authors and the co-corresponding author, Mabel, gives us some insights into the story behind the paper.
What was already known about the topic?
Tissue culture-induced de novo shoot regeneration is one of the many remarkable regenerative abilities of plants. It relies on the right balance of phytohormones, auxin and cytokinin, to exploit the totipotency of plant cells and generate entire shoot and/root systems from any tissue. High auxin promotes the formation of undifferentiated callus from which shoots arise. Notably, only a few cells of the callus could reprogram and develop into a complete shoot system (Gordon et al., 2007; Kareem et al., 2015).
How did you get started on this project?
We called the sub-population of callus cells that have the potential to make the shoot ‘progenitors’. These cells were marked with the expression of polar auxin efflux carrier, PINFORMED1 (PIN1). We were curious about the stochastic selection of certain cells to become progenitors and how they progress into the shoot (Fig 1A). To understand this, we followed hundreds of progenitors in real-time by confocal-based live imaging and found that around one fourth of these progenitors did not make shoot meristem. Why some sub-populations succeeded in making shoot meristem while others failed intrigued us. This was where the project started.
When doing the research, did you have any particular result or eureka moment that has stuck with you?
Contrary to popular expectation, our investigations revealed that the reason why some progenitors aborted was not the lack of the shoot stem cell regulator, WUSCHEL. The observation was striking; even though WUS is necessary (Zhang et al., 2017), its abundance alone does not guarantee successful shoot regeneration. Rather it was the localization pattern of cell polarity markers such as PIN1, that predicted successful shoot regeneration. The next goal was to identify the mechanism by which these progenitors achieved shoot regeneration. We performed a comparative transcriptome analysis using several genetically engineered backgrounds; some of which could regenerate shoot and others that could not. While profiling the changes in gene expression during the onset of progenitor formation, we identified an over-representation of XYLOGLUCAN ENDOTRANSGLUCOSYLASE/HYDROLASE 9 (XTH9) in the backgrounds that could regenerate shoot. XTH9 encodes an enzyme for cell wall loosening and had an unexpected spatial expression. We found it to be expressed solely in a shell of cells (which we refer to as the non-progenitor cells) encapsulating the progenitor, that underwent stretching. As opposed to the commonly held notion that stretching cells often divide, these surrounding cells hardly divided. This was a hypothesis-generating result and turned out to be a key milestone for the story.
What was the key experiment?
We were curious about what causes the specific local expression of XTH9. Through extensive follow-up genetic and biochemical approaches including ChIP seq, we discovered that a transcription factor and shoot-promoting factor, CUP-SHAPED COTYLEDON 2 (CUC2), activated XTH9 expression solely in non-progenitor cells. We further established that the CUC2-XTH9 regulatory axis promoted cell polarity in the progenitor non-cell autonomously. In parallel, Anju modulated the components of the regulatory axis with the challenging inducible system, which allowed us to capture even their temporal necessity and their transient behavior. Thus, we were able to identify the biochemical component that conferred productive fate to the regenerating progenitors. This exciting result made us even more intrigued to investigate how the coordination between the progenitor and its neighbors is able to generate the precise biochemical output for shoot regeneration. We asked what is the nature of this coordinated interaction between the progenitor and neighboring non-progenitor cells? What happens if you disrupt it? To answer this, we undertook a series of approaches. We tracked the growth of the progenitor cells and the neighboring non-progenitor cells at single-cell resolution. Then, we analyzed their differential growth rate using MorphoGraphX. We studied the differential stress patterns between the progenitor cells and their neighbors by visualizing their microtubule orientation. And finally, we disrupted the coordination by targeted laser ablation of either the progenitor or their neighboring cells. These key experiments led us to the following two conclusions: First, there is a mechanical conflict between the progenitor cells and their neighbors. Second, feedback between mechanical and biochemical properties of the cells is crucial to self-organize the cells of shoot progenitors in the absence of any tissue patterning cues. By this time, it was clear that we needed a model to interpret these conclusions. We proposed four models and eliminated three of them. It was most exciting as we steered closer to that one model that all our experimental evidence aligned with. Through this model, we proposed that the expanding non-progenitor cells act as a ‘constriction shell’ similar to a rubber band serving a dual role. First, to facilitate the enclosed progenitor cells to grow and divide, and second to provide a mechanical constriction causing the progenitor cells to bulge out. Meanwhile, the growth of the progenitor cells likely feeds back on the non-progenitor and further triggers its expansion (Fig. 1B-1C) (Varapparambath et al., 2022).
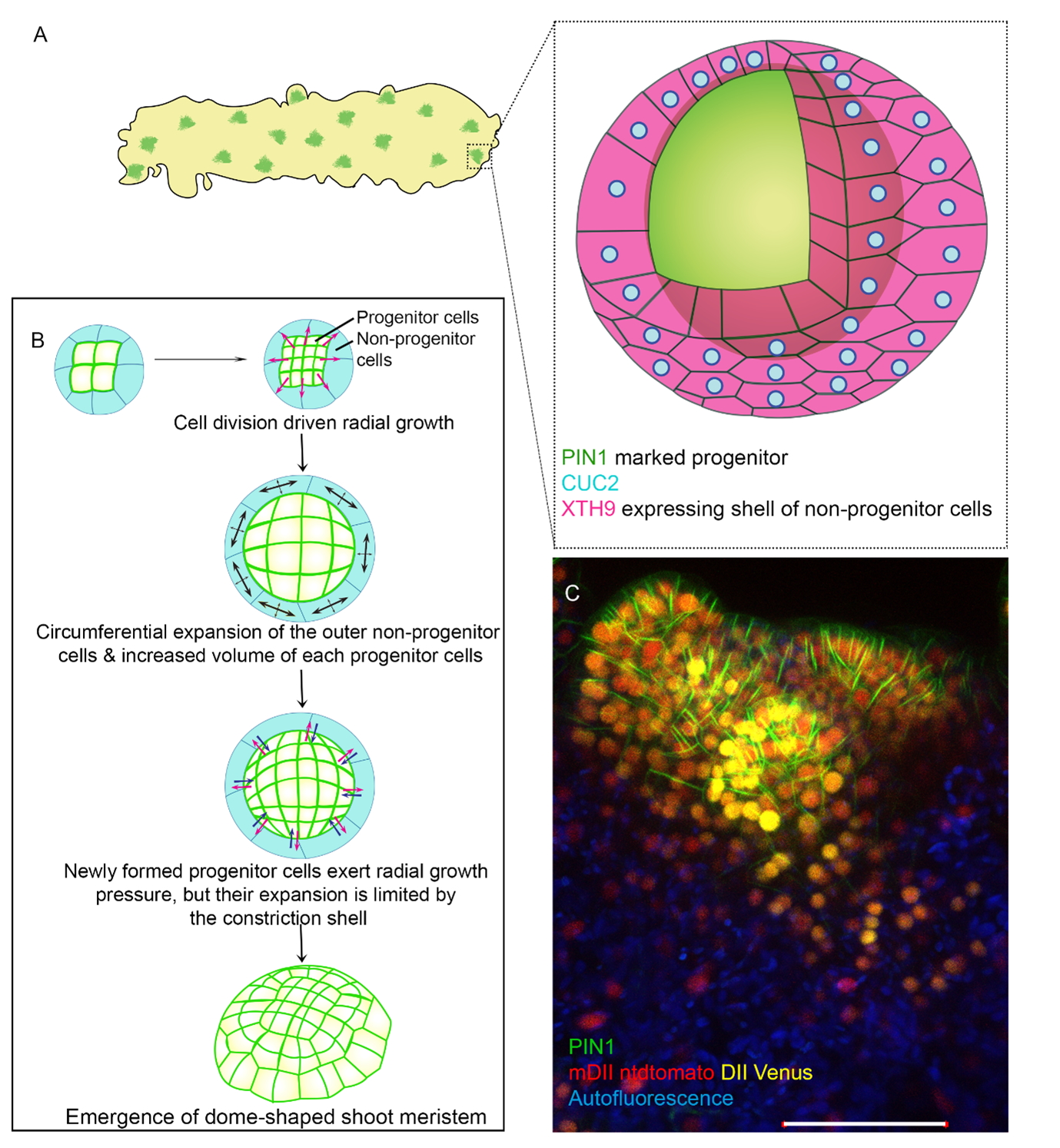
And what about the flipside: any moments of frustration or despair?
The progenitors, during their early stages, will be buried beneath 2-3 layers of callus cells which makes the progenitor detection and their real-time tracking challenging. This, in addition to the irregular topology of the callus, makes it easy to miss the progenitors. But unfortunately, that was not all. We all could uniformly agree that after performing a whole genome transcriptome approach, you will always end up with more than what you need. This happened to us as well. After much struggle and a marathon of efforts by one of the co-first authors, Vijina, to lead several follow-up genetic experiments and ChIP seq, we landed on a single target, XTH9.
Where will this story take the lab?
This is the first study to integrate the feedback between tissue mechanics and biochemical pathways for specifying cell identity during plant regeneration. But that is just the tip of the iceberg. The lab’s long-term goal will be to seek answers to some of the fundamental questions such as the link between cell division and cell polarity during de novo organogenesis. The lab is also in the process of branching out into exploring cellular heterogeneity using de novo shoot regeneration as a model.
What is next for you after this paper?
After working on this story, I developed an inclination toward the relationship between mechanics, cell polarity, and cell fate. I look forward to exploring it further through theory and modelling-based approaches. Co-first author Vijina aspires to step into the field of evolutionary development. The other co-first author, Anju has her mind fixed on delving deeper into the cell biology of fundamental life processes not just in plants, but also in other organisms.
References
Gordon, S. P., Heisler, M. G., Reddy, G. V, Ohno, C., Das, P. and Meyerowitz, E. M. (2007). Pattern formation during de novo assembly of the Arabidopsis shoot meristem. Development 134, 3539–3548.
Kareem, A., Durgaprasad, K., Sugimoto, K., Du, Y., Pulianmackal, A. J., Trivedi, Z. B., Abhayadev, P. V, Pinon, V., Meyerowitz, E. M., Scheres, B., et al. (2015). PLETHORA Genes Control Regeneration by a Two-Step Mechanism. Curr Biol 25, 1017–1030.
Varapparambath, V., Mathew, M. M., Shanmukhan, A. P., Radhakrishnan, D., Kareem, A., Verma, S., Ramalho, J. J., Manoj, B., Vellandath, A. R. and Aiyaz, M. (2022). Mechanical conflict caused by a cell-wall-loosening enzyme activates de novo shoot regeneration. Dev. Cell 57, 2063–2080.
Zhang, T.-Q., Lian, H., Zhou, C.-M., Xu, L., Jiao, Y. and Wang, J.-W. (2017). A Two-Step Model for de Novo Activation of <em>WUSCHEL</em> during Plant Shoot Regeneration. Plant Cell 29, 1073 LP – 1087.