Conversations with my parents (about adult chondrogenesis and spontaneous cartilage repair in the skate, Leucoraja erinacea)
Posted by andrewgillis, on 23 June 2020
One night, during the summer of 2012, I found myself sitting in a cottage in Woods Hole, trying to explain to my parents why I’d spent much of my professional life studying the little skate (Figure 1). I was a postdoctoral fellow at Dalhousie University at the time, and working almost exclusively with skate as a model system was hard: I could only access animals while visiting the Marine Biological Laboratory (Figure 2), and while I was fortunate enough to spend my summers in Woods Hole (thanks largely to generous support from the MBL Whitman Center Fellowships programme and the MBL Embryology Course), there were relatively few tools and resources available for the skate at that time, and limited access to animals meant that I was under a great deal of pressure to design, optimise and complete experiments during a relatively short time window each year. Why would anyone do this to themselves?!
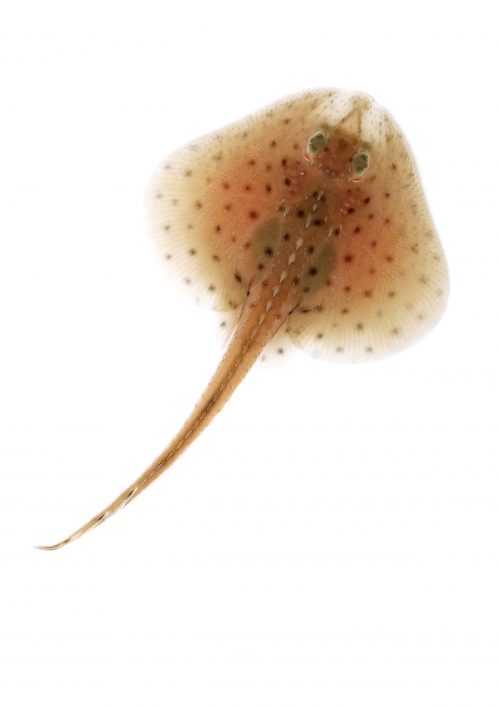
Up until that point, I’d worked exclusively on skate embryonic development. I explained to my parents that skates occupied a very important position in vertebrate evolutionary history, and that by studying skate embryos, and comparing our findings with what we know from work in mouse, chick, frog and zebrafish, we are able to make inferences about anatomical features (and developmental mechanisms) that were present in the last common ancestor of jawed vertebrates. That’s a perfectly good and valid reason for studying skate embryos, isn’t it? So why was I getting the “blank look” that many of us scientists know and dread? Could it be that aspiring to infer ancestral developmental mechanisms, while a perfectly good and reasonable academic endeavour, is not necessarily the most compelling reason for studying an obscure marine organism, in the eyes of a non-scientist? Like a stand-up comic squaring off against a heckler, I felt the need to come up with something else – and fast.
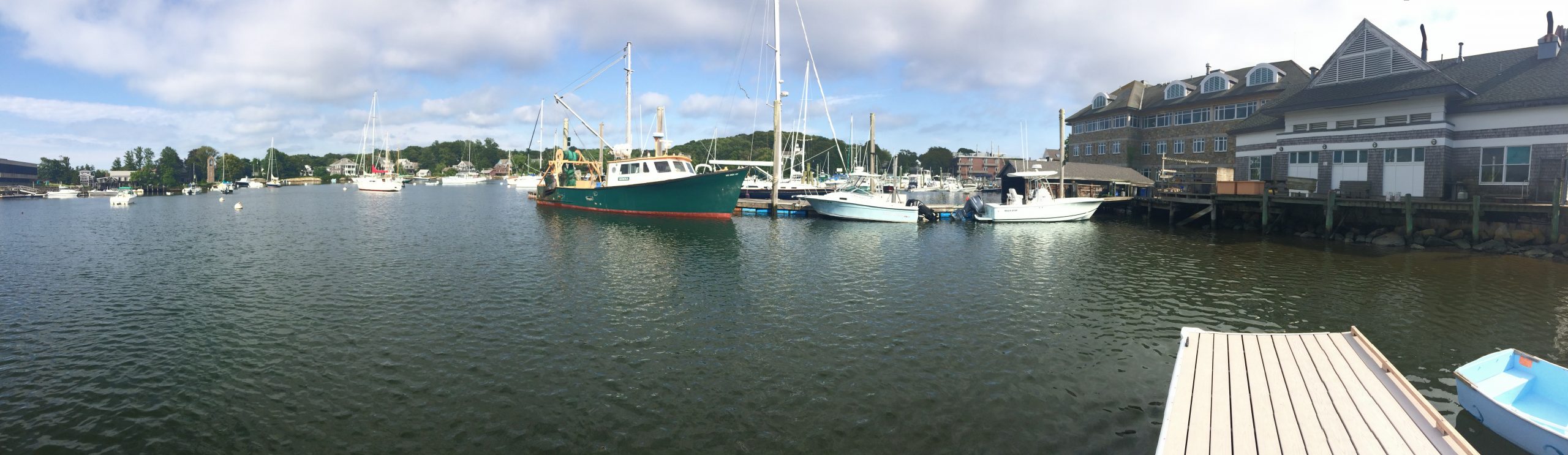
Sometimes, such moments of forced introspection push us to recognise things that we’ve long known, but rarely acknowledge. I started to think about how, for as long as I could remember, I’d been drawn to the sea, and fascinated by the amazing diversity of form that exists within it. Many marine organisms, with their remarkable (and, sometimes, downright bizarre) adaptations, have arrived at solutions to seemingly intractable biological problems – and if I was being honest, I was just as interested in understanding the skate’s oddities as I was in resolving deeply conserved features that were present at the origin of jawed vertebrates.
So, what’s weird and wonderful about the skate? Hmmmm, flat as a pancake? Electroreceptive? Toothy denticles on the skin? Oviparous mode of reproduction? I’ve always worked on skeletal development, and both my parents suffer from arthritis, and so, in that moment, I pivoted to my go-to organ system – the skeleton – and grasped at three relatively simple observations. The first observation was that cartilaginous fishes (the group of animals that includes sharks, skates and stingrays) do not form bone, but rather (as their name suggests), possess a skeleton that is composed almost entirely of cartilage. This stands in stark contrast to what is seen in mammals: in mammals, cartilage is predominantly an embryonic tissue, and the vast majority of cartilage is replaced by bone, through a process known as endochondral ossification. In adult mammals, cartilage persists in very few places within the skeleton – among them, as the thin pads of articular cartilage that are found within joints. The second observation was that, although cartilage is a rather homogeneous and simple-looking tissue (it only has a single cell type – the chondrocyte – Figure 3), it has very little capacity for spontaneous repair (for example, following articular cartilage damage), and it is surprisingly difficult to engineer in vitro. While much progress has been made in developing cell-based therapies for cartilage repair, many of these approaches are still hindered by the frustrating tendency of in vitro cartilage to differentiate toward a bony fate. The third observation related to the old adage that your pet goldfish will grow to the size of its tank. Of course, this is a gross oversimplification, but it is generally true that, unlike mammals (whose growth curves plateau), many fishes do, indeed, continue to grow (albeit very slowly) in a largely indeterminate manner.
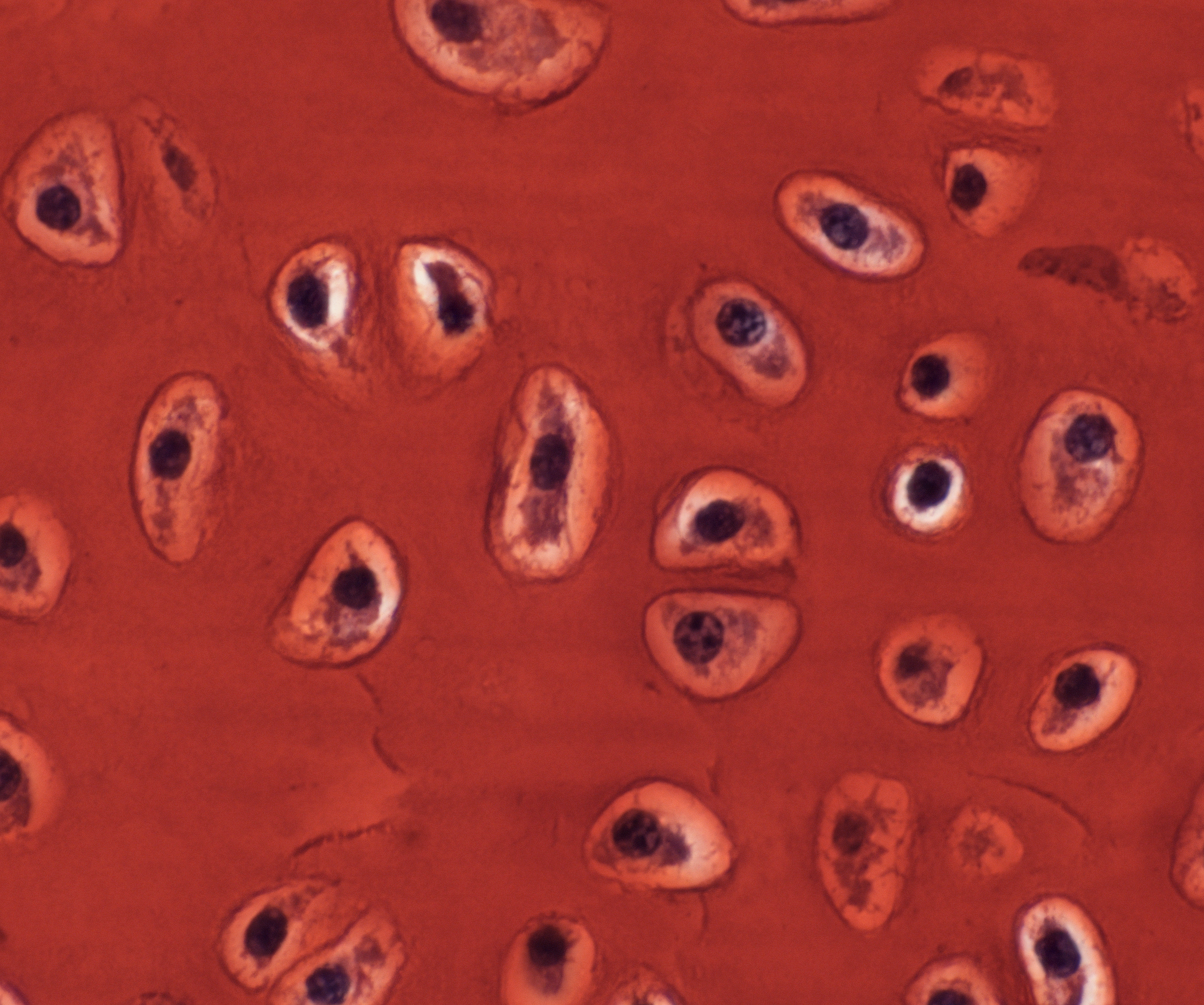
And so, I pondered out loud, if the skate skeleton is composed entirely of cartilage, and if that skeleton exhibits some sort of indeterminate growth, could it be that skates hold, within their skeleton, a mechanism to generate new cartilage in adulthood? And could this mechanism not only allow them to generate new cartilage during normal growth, but perhaps also to repair injured cartilage? There is mounting evidence that the cartilaginous skeleton of sharks and skates is a derived condition – in other words, cartilaginous fishes didn’t primitively lack bone, but rather lost it (for the latest on this topic, see this exciting pre-print by Martin Brazeau, Sam Giles and colleagues). If, through evolution, cartilaginous fishes have come up with a way to make cartilage as a permanent, growing tissue (rather than as an embryonic stepping stone toward bone) – and if they did so from an ancestral bony condition – could studies of cartilage development, growth and repair in skate inform new approaches to cartilage repair in mammals? Needless to say, at this point, my parents were rapt, sold, and ready to get in on the ground level…
While it is absolutely true that my lab’s work on cartilage growth and repair was born from a conversation with my parents, it is an exaggeration to say that this project crystallised and rolled out as smoothly as I set out above… In reality, after that fateful conversation, this project came together very episodically, over nearly 8 years (and mainly as a side project). Below, I’ll briefly run you through some of the major findings of our recent paper on adult chondrogenesis and cartilage repair in the skate (in the order in which they occurred – which, probably unsurprisingly, is not the order in which they appear in the paper…). In many cases, the figures that I’ve included here show more detail than I can succinctly address in the text – so for further information, please do take a look at our paper.
The fin cartilages of adult skates are permeated by canals, containing blood vessels and other cell types
In the 1980’s, it was reported that the vertebral cartilages of some large sharks were permeated by a series of canals (Hoenig and Walsh, 1982, Can. J. Zool. 60: 483-485). This isn’t something that is seen in the embryonic cartilages of other vertebrates (except for the transient invasion of cartilage by canals during endochondral ossification), and so it was speculated that these canals could function in the nourishment, maintenance and growth of cartilage in these sharks during adulthood. We decided to focus our study on the metapterygium (one of the basal fin cartilages) of the skate, as this element is easily recognisable at all life stages, and its location makes it easily accessible for surgical manipulation (Figure 4). We cut sections through the metapterygium of adult skates, and saw that this element is also permeated by cartilage canals. These canals originate in the perichondrium (the fibrous tissue that wraps around the outside of cartilaginous elements), and extend into the core of the metapterygium. In agreement with earlier reports, we found that these canals contained blood vessels as well as a number of other connective tissue-like cell types, and we found that the blind ends of these canals were sites of active deposition of type II collagen (the predominant collagen of cartilage extracellular matrix) (Figure 5). Could the perichondrium be a source of cartilage progenitor cells in adult skates? And could these canals serve as a means of delivering cells and generating new extracellular matrix deep within the cartilage of the metapterygium? Testing for label retaining cells within the adult skate skeleton, and attempting to trace their progeny, would have been a logical next step – and we would eventually get to that – but not before first asking…
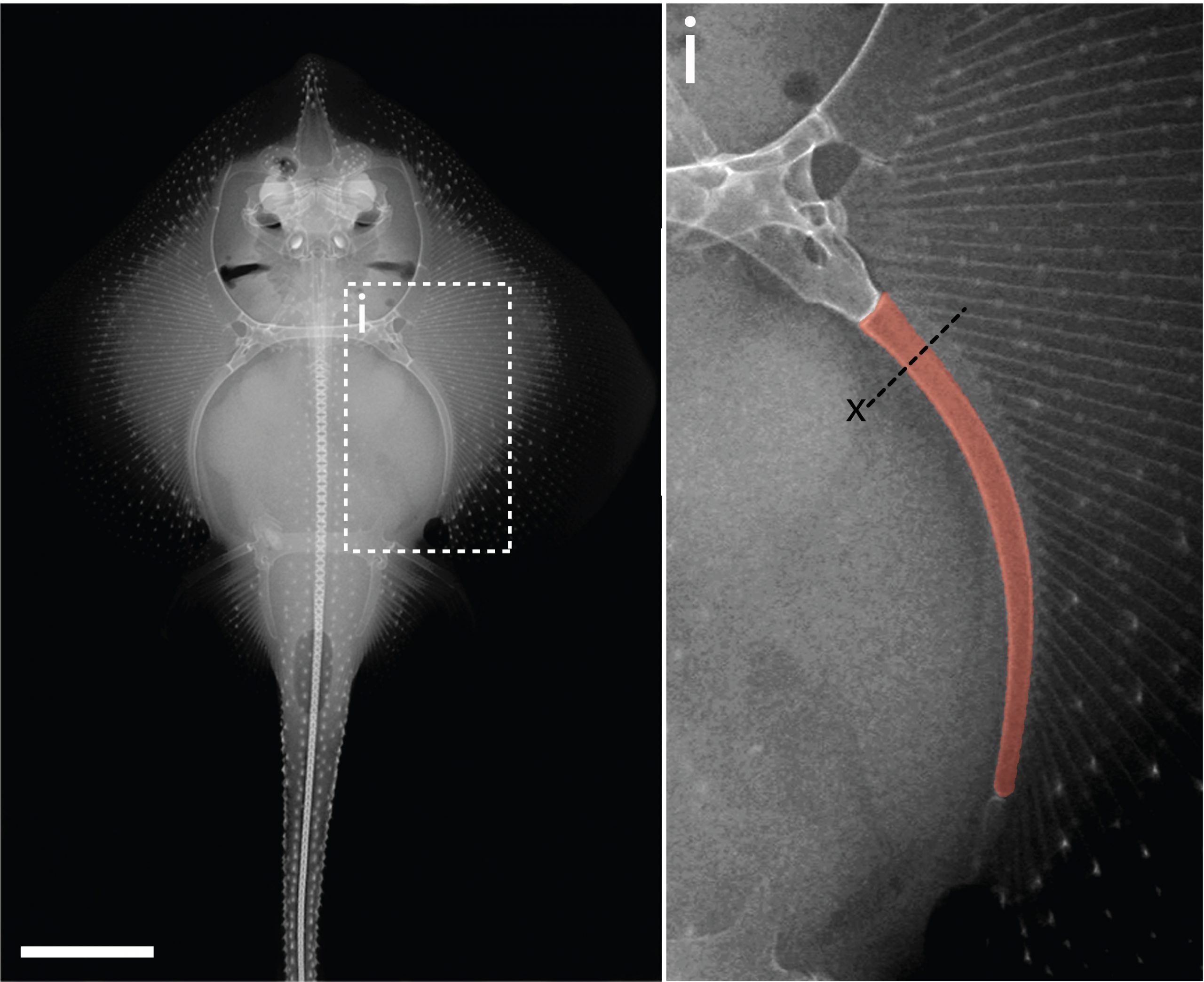
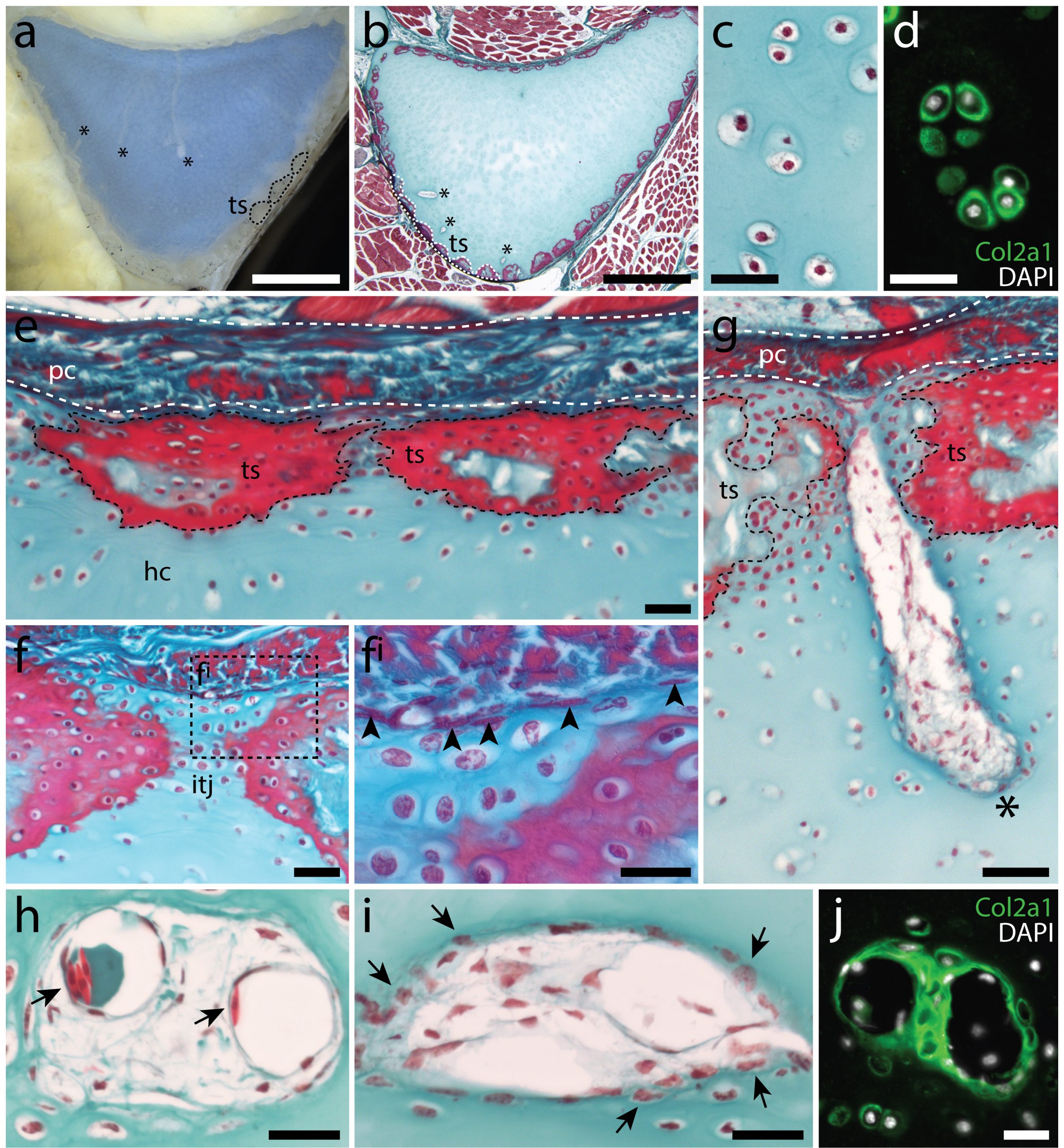
Can skates can spontaneously repair injured cartilage?
Why not shoot straight for the moon, right? I was interested in testing whether adult skates could spontaneously repair a cartilage injury that was comparable in scale to a very severe mammalian osteoarthritic lesion. The problem was, I had no money to carry out these experiments (adult skates require a large amount of tank space and care), and no experience working with adult skates. So I decided to apply for a Research Grant from the Fisheries Society of the British Isles (which would just cover the costs of animal care), and after a few failed attempts, I was eventually successful. I also joined forces with then MBL veterinarian, Dr. Amy Hancock-Ronemus, who had a lot of experience with the maintenance and care of adult skates, and who was keen to collaborate on this work. Amy and I developed a biopsy protocol to remove a ~3-4mm wedge of cartilage from the metapterygium of adult skates, and we performed this procedure on twenty-six animals, collecting two animals shortly after the procedure, and then two animals per month for the following year, to assess repair.
Mammalian articular cartilage injuries cannot spontaneously repair – rather, injury sites become filled with scar tissue, or with fibrocartilage, which is mechanically inferior to the hyaline cartilage that typically resides in joints. In skate, we noticed that at 2 months post-operation, the cartilage injury sites that we induced in the metapterygium were completely filled with an undifferentiated connective tissue, and that by 3 months, this tissue began to differentiate into hyaline cartilage. Histologically, this repair cartilage resembled adjacent uninjured cartilage – it contained chondrocytes, and an extracellular matrix composed of type II collagen that was continuous with the matrix of adjacent non-injured cartilage. This repair tissue progressively differentiated from the bottom of the injury site to the top, and by 11-12 months post-operation, the injury sites were completely filled with new cartilage (Figure 6). These observations suggested to us that skates do have the capacity to spontaneously repair injured cartilage, though they didn’t tell us where the repair tissue comes from. However, by chance, a small number of our cartilage biopsies were not completely successful, resulting only in damage to the perichondrium, without removal of a wedge from the underlying cartilage. In these cases, we observed the formation of a large mass of new cartilage between the native cartilage and the perichondrium. This led us to hypothesise that the perichondrium was the source of new cartilage during the skate’s injury repair response, and that mechanical disturbance of the perichondrium, even without the removal of underlying cartilage, was sufficient to trigger the onset of chondrogenesis.
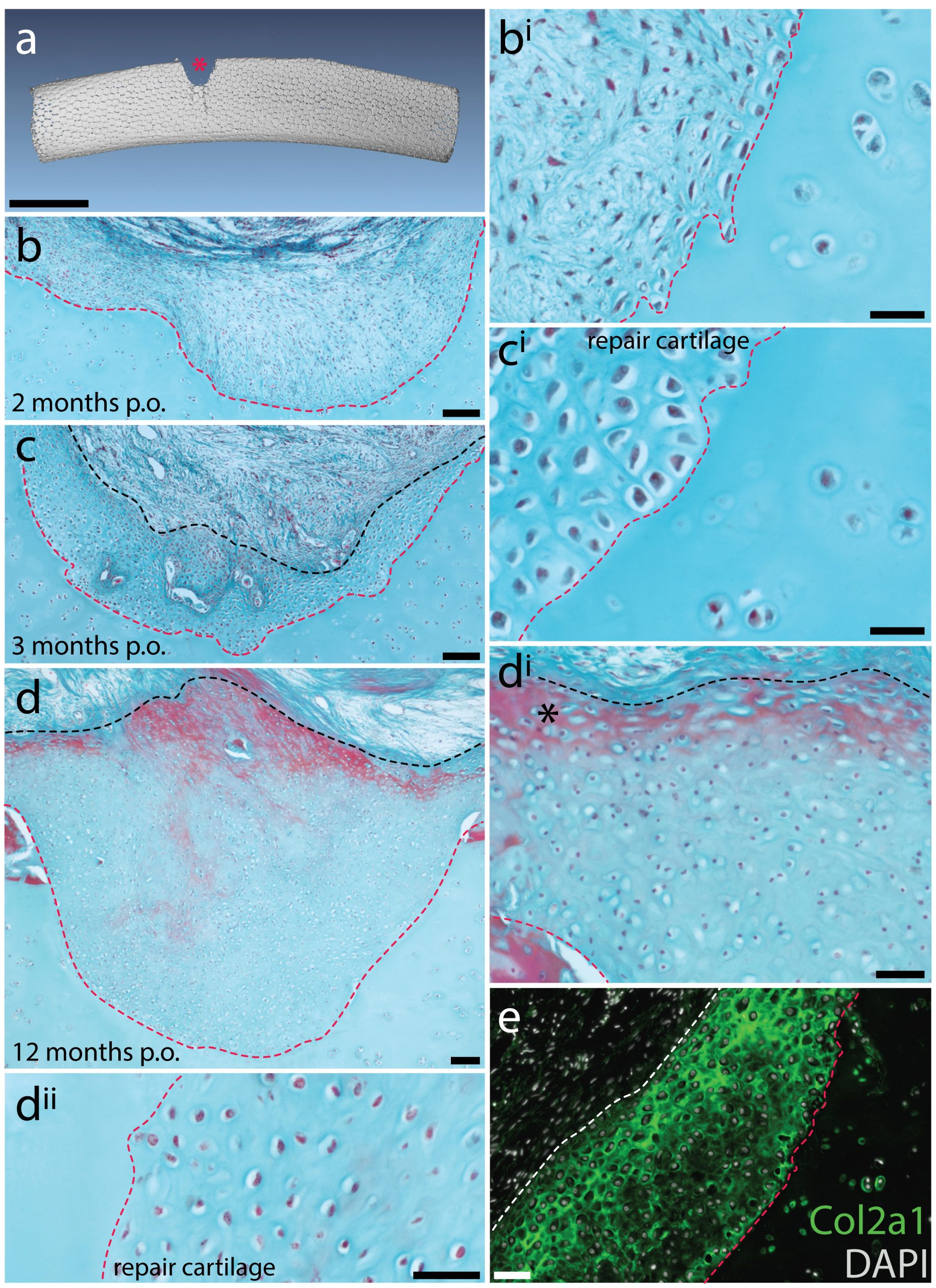
Skates possess label-retaining progenitor cells in their perichondrium, and these cells give rise to new chondrocytes in adults
Label retention experiments have long been used in the fields of cell/developmental biology and regeneration, as a means of testing for the presence of dividing cells (for example, putative progenitor cells) in vivo. Briefly, a label that will become incorporated into newly synthesised DNA is introduced into a system, and this label is then detected and visualised, with retention used as a proxy for cell division. Historically, tritiated thymidine or bromodeoxyuridine (BrdU) has been used for such experiments, though 5-ethynyl-2-deoxyuridine (EdU) has emerged as an increasingly popular alternative because of the relative ease, speed and simplicity of its detection. We conducted an EdU retention experiment in adult skates, with the aim of resolving whether/where cycling cells (i.e. putative progenitor cells) were located within the adult skeleton. We gave adult skates 3 intraperitoneal injections of EdU (with each injection spaced two days apart), and then we tested for the presence of EdU-retaining cells in the metapterygium. We noted that EdU-labeled cells were recovered exclusively within the perichondrium, with no label-retaining cells in the cartilage itself. We also noticed two morphologically distinct populations of EdU-positive cells within the perichondrium – the “outer” perichondral cells, with round nuclei, and the “inner” perichondral cells, which have flattened nuclei, and which sit at the boundary between the perichondrium and the underlying cartilage. These observations suggested to us that cartilage growth in adult skates likely involved a population of progenitor cells within the perichondrium, and wasn’t occurring through continuous proliferation of differentiated chondrocytes.
We reasoned that 1) as cell division was likely quite slow in the skate, 2) as EdU remains detectable in the progeny of labelled cells for several rounds of division, and 3) as label-retaining cells were located only within the perichondrium at the time of injection, we might be able to use this labelling approach to lineage trace these putative perichondral progenitor cells. So we conducted a set of EdU pulse-chase experiments, this time collecting animals at 1, 2, and 5.5 months post-injection – and this is where Aleksandra Marconi joined the project. By this time, I’d left Dalhousie University and started my own group in the Department of Zoology at the University of Cambridge. Aleks is a PhD student on our Wellcome-funded developmental biology PhD programme, and was interested in the problem of post embryonic cartilage growth in the skate. Aleks decided to do a rotation in my lab, and was keen to analyse the adult skate label retention experiments that had been sitting on my shelf for a couple of years. After confirming the presence of EdU-positive cells within the perichondrium of the metapterygium at the time of EdU injection, Aleks found that by 2 months post-injection, she began to see an increase in the number of EdU-positive cells within the perichondrium, and the appearance of EdU-positive cells within the cartilage canals. And by 5.5 months post-injections, she recovered EdU-positive cells within the perichondrium and cartilage canals, as well as EdU-positive chondrocytes at two places within the cartilage of the metapterygium: superficially, beneath the perichondrium, and deeper within the cartilage, adjacent to the blind-end of cartilage canals (Figure 7). These observations led us to propose that the perichondrium of the adult skate metapterygium houses a population of (likely self-renewing) label-retaining progenitor cells, and that these progenitors give rise to new cartilage throughout adulthood via two routes: superficially, by apposition from the perichondrium, and interstitially, via transport of progenitor progeny within cartilage canals.
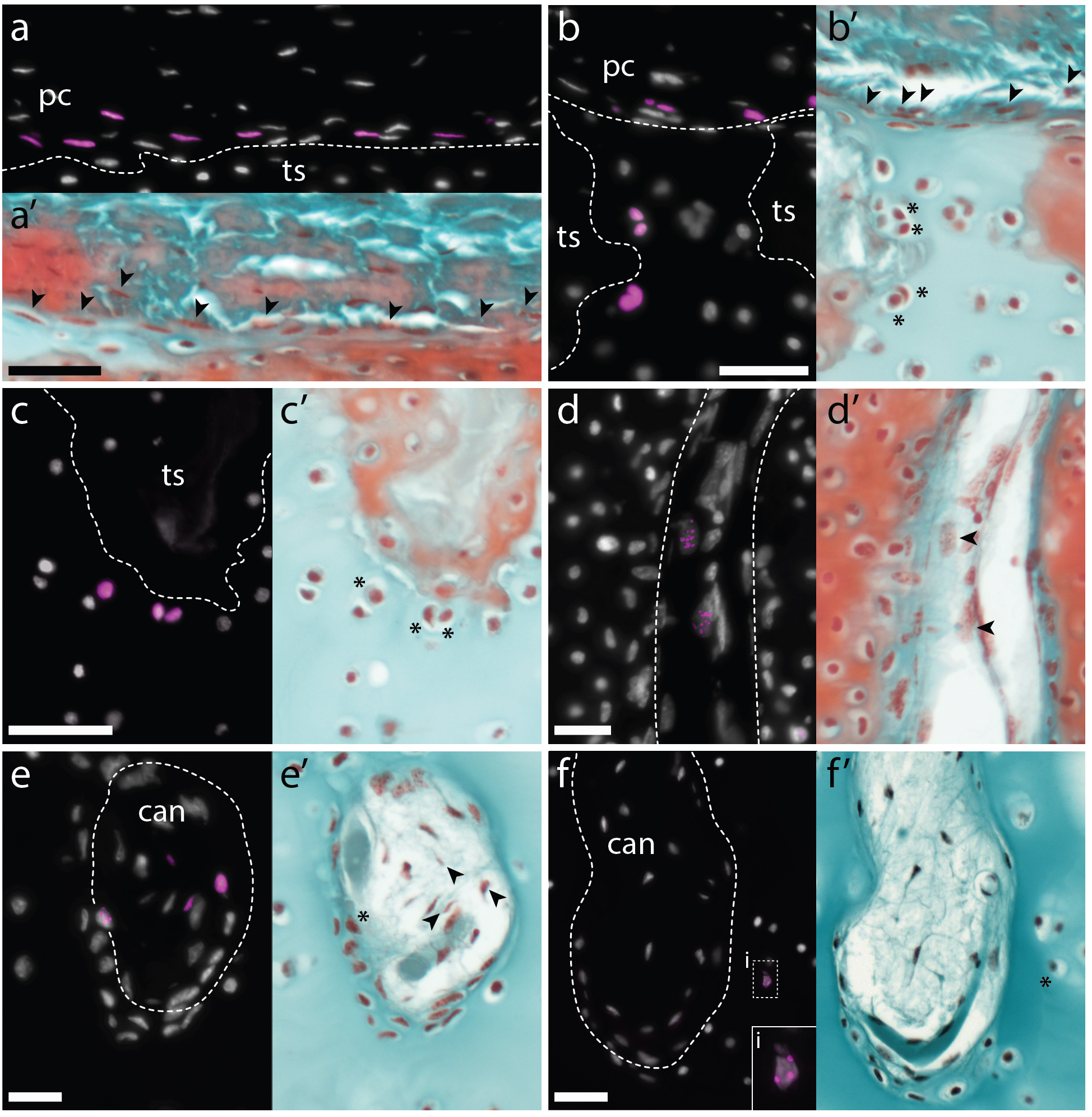
Skate inner perichondral cells express markers of embryonic chondrogenesis
In parallel with the above experiments, we were also looking at gene expression within the embryonic, juvenile and adult skate skeleton. Around that time, we began studying gene expression by hybridisation chain reaction (HCR) – a simple and sensitive method for multiplexed fluorescent mRNA in situ hybridisation, in any taxon, and with any tissue prep (Choi et al., 2018, Development, 145: dev165753). In mammals, differentiation of mesenchymal cells into chondrocytes is marked by the expression of Col2a1 (the gene encoding type II collagen), and Col2a1 is directly transcriptionally regulated by the SoxE and SoxD-class transcription factors Sox9 and Sox5/6. We found that, as in mammals, embryonic chondrocytes in the skate co-expressed Col2a1, Sox9, Sox5 and Sox6, pointing to the likely conservation of this cartilage gene regulatory mechanism across jawed vertebrates (Figure 8). Additionally, within the juvenile and adult skeletons, we found that Col2a1, Sox9, Sox5 and Sox6 were predominantly expressed in the periphery of the cartilage (consistent with our model of appositional growth, and the birth of new chondrocytes around the periphery of the metapterygium), and we noticed that the morphologically distinct “inner perichondral cells” (i.e. the flattened cells that sit at the boundary between the perichondrium and underlying cartilage, and that were identified as putative progenitor cells in our label retention experiments) express Sox9, Sox5 and Sox6, but not Col2a1 (Figure 9). This is consistent with the hypothesis that these cells have chondrogenic potential, but have not yet differentiated into chondrocytes.
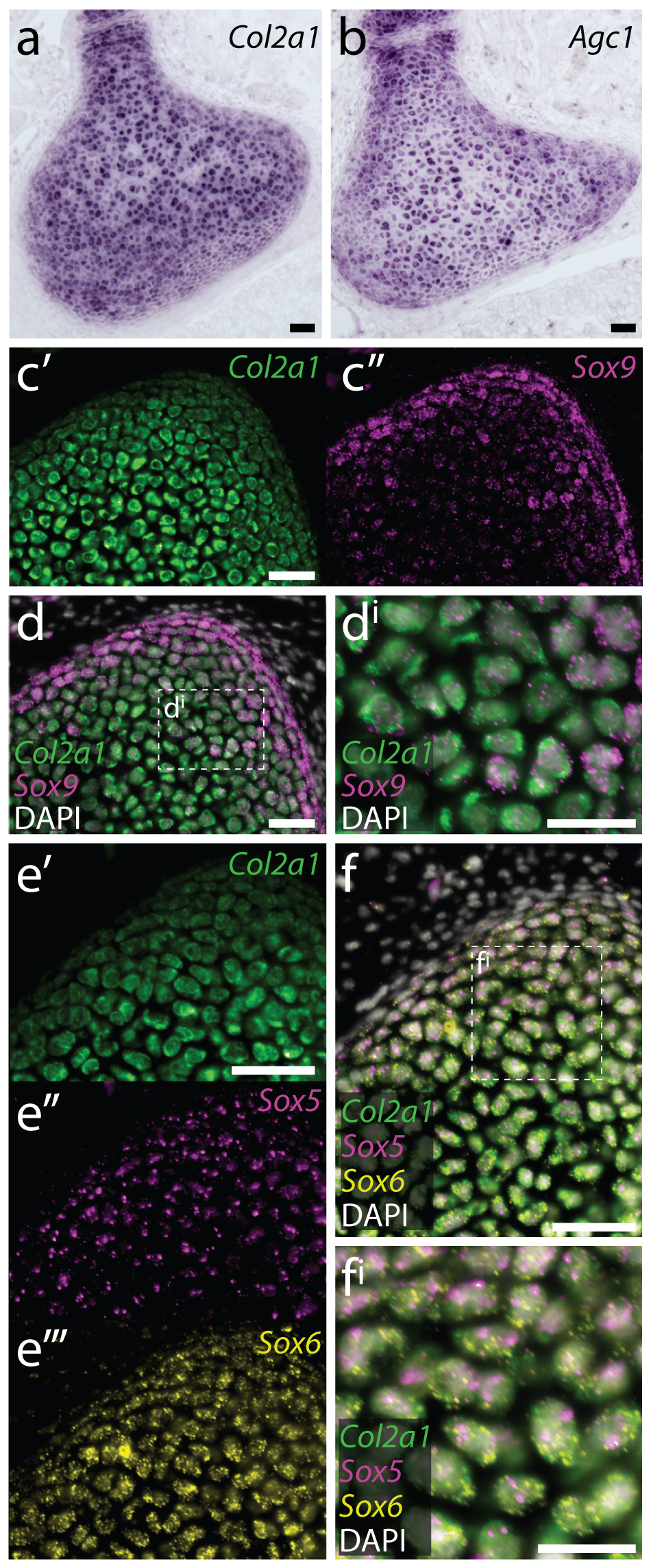
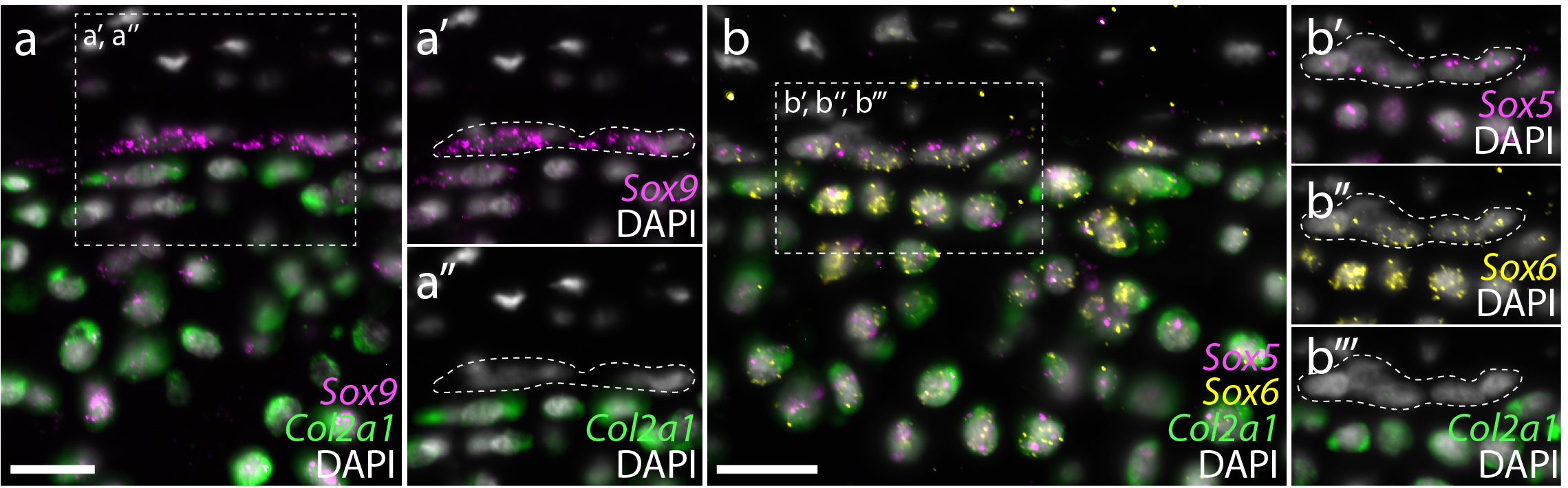
We therefore propose that post-embryonic growth of cartilage in the skate occurs by the slow-but-steady development of new chondrocytes from a population of perichondral progenitor cells, and that these progenitor cells are recognisable, transcriptionally, by their expression of the pro-chondrogenic transcription factors Sox9, Sox5 and Sox6. We also show that the persistent presence of perichondral progenitor cells in adult skates correlates with an ability to spontaneously repair injured cartilage. We think that these are really exciting findings, though, of course, there are still a number of outstanding questions: Is the perichondrium homogeneous in its chondrogenic potential? Or, is there a specialised subpopulation of perichondral chondroprogenitor cells? To what extent is the endochondral ossification transcriptional programme conserved in cartilaginous fishes? And where does this programme arrest to permit the persistence of cartilage as a permanent skeletal tissue? Our work toward answering these questions is ongoing, and we look forward to sharing more of our progress with the developmental biology and regeneration community shortly.
So, what started off as a side project, inspired by a conversation with my parents and supported initially by a small society grant, has now become a major new line of research in my lab, and has renewed my curiosity in (and enthusiasm for) the bizarre little skate that I started working on as a graduate student fifteen years ago. Moreover, I think that this project illustrates a point that is made most eloquently by a quote attributed to the former MBL scientist, Nobel laureate Albert Szent-Gyorgi (and that is emblazoned on the wall leading into the tank room at the MBL Marine Resources Centre): “Research is to see what everybody else has seen and think what nobody has thought”. This research project stemmed from three observations – none of which were particularly novel or profound (or even our own to begin with!). But by digging a little deeper and conducting a few simple experiments, we were nevertheless able to learn something new and exciting about the unique skeletal biology of cartilaginous fishes.
As a side note: in 2021, my lab will be relocating to the Marine Biological Laboratory in Woods Hole, where I will be taking up a resident scientist position. I am very excited about this move, not least because it will allow us to develop the skate even further as a model system for developmental biology and regeneration. And I recently learned from my friend, colleague and fellow Woods Hole enthusiast, historian of science Shane Jinson, that the lab space we are moving into in the Lillie Building once belonged to, of all people, Albert Szent-Gyorgi (Figure 10)!
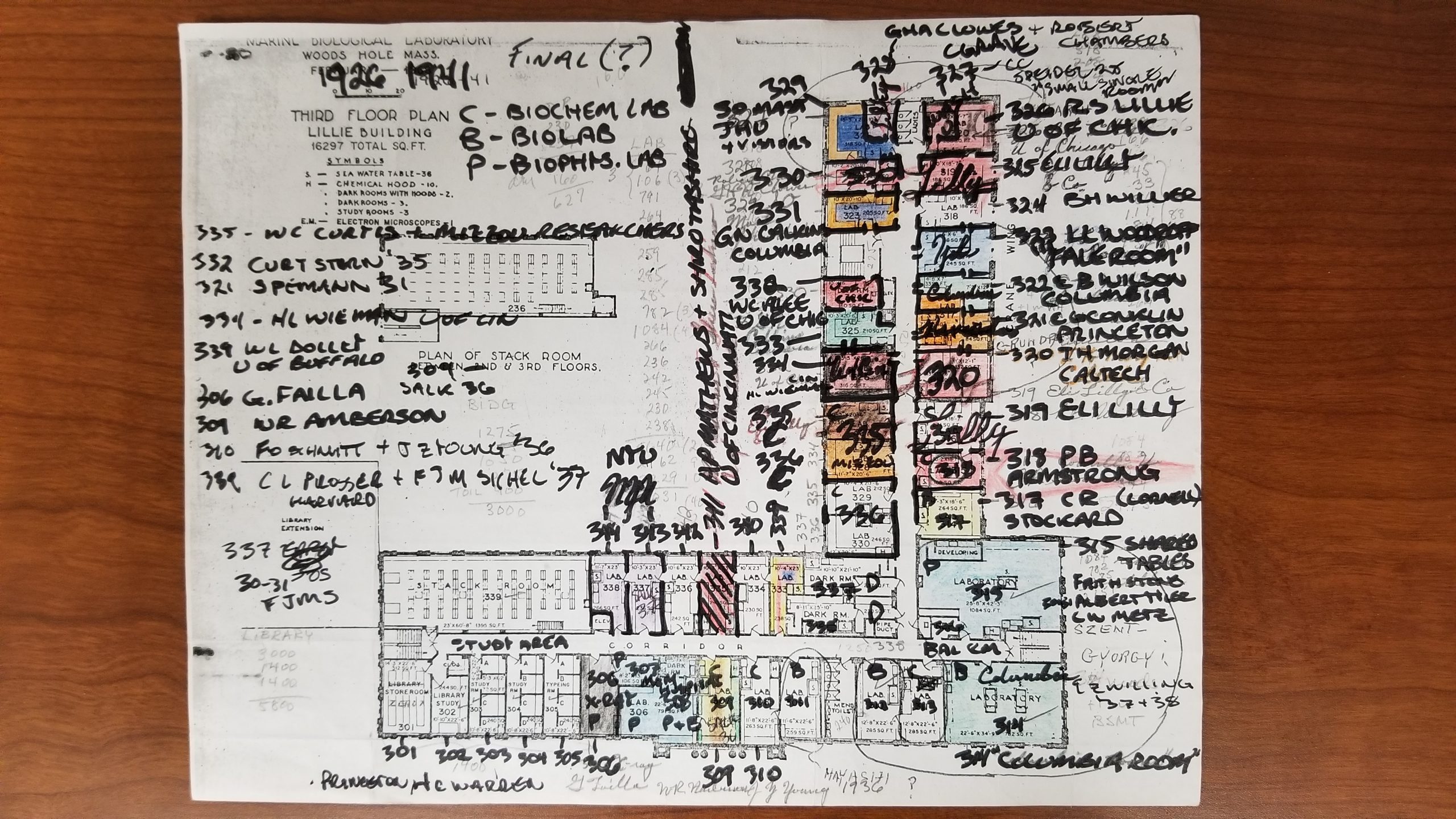
Congratulations on this elegant study and good luck with your move!