Down from the crest to clear the dead
Posted by SKucenas, on 30 September 2019
Yunlu Zhu & Sarah Kucenas
Introduction
Cells are the building blocks of life. However, in multi-cellular organisms, millions of cells are subject to death due to injury, infection and ordinary cell turnover (Galluzzi et al., 2018). For example, epithelial cells in the small intestine rapidly renew every 2 to 6 days in most mammals, which is crucial to maintain proper function of villus epithelia (Mayhew et al., 1999). Moreover, during embryonic development, cell death serves as a crucial mechanism to remove unnecessary cells, adjust tissue size and shape, as well as correct developmental errors (Arya and White, 2015). In order to maintain homeostasis and avoid unwanted inflammatory responses, cellular debris is usually cleared rapidly by professional phagocytes, such as macrophages and microglia. However, during early neurogenesis when the neural tube develops and large numbers of neurons and glia undergo apoptosis, myeloid-derived professional phagocytes have not yet infiltrated the trunk of developing embryos (Herbomel, Thisse and Thisse, 1999, 2001; McGrath et al., 2003; Bertrand et al., 2013; Stremmel et al., 2018). How dead cells are removed from this region during early development remained largely unknown until recently.
In our recent paper entitled “Migratory Neural Crest Cells Phagocytose Dead Cells in the Developing Nervous System”, we demonstrate an unexpected behavior of neural crest cells (NCCs), which have been studied for more than 150 years, in debris clearance before the colonization of professional phagocytes (Zhu et al., 2019) (Figure 1).
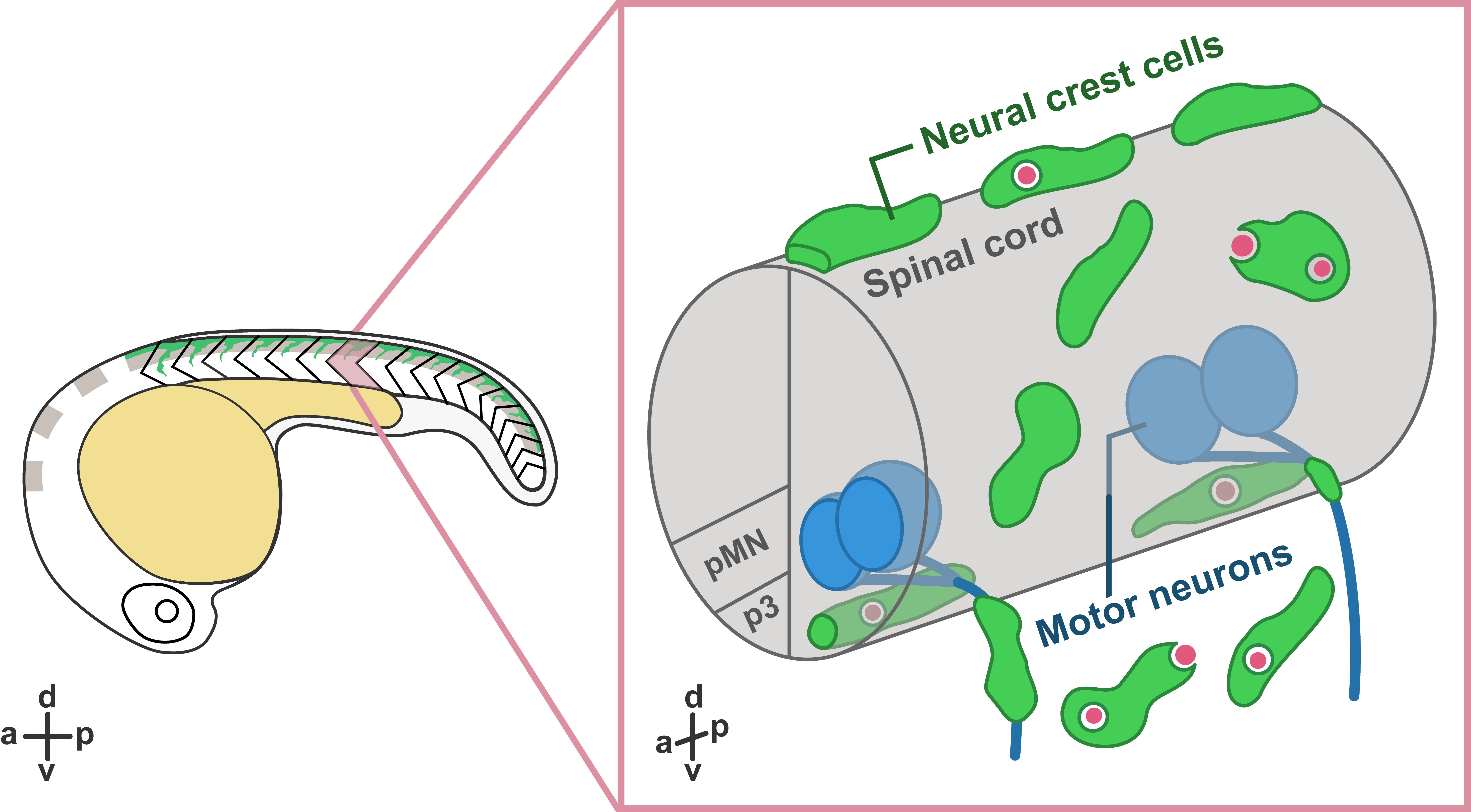
Interestingly, not only did we not expect this novel function of NCCs, but the discovery was also made by accident! Our original intent was to study how glial populations coordinated their behaviors during early spinal motor nerve development. But early in our studies, Yunlu started to notice NCCs behaving in a manner that didn’t match what the literature described. Some migrated away from their migratory streams, and others appeared to interact with cellular debris. Here, we would like to share the story behind this work.
Neural Crest Cells Clear Debris
What are NCCs and what do we know about them? During the earliest stages of vertebrate nervous system development, the ends of neural plate rise and fold into the neural tube, which further develops into the central nervous system (CNS), including the brain and the spinal cord. During or after the closure of the neural tube, NCCs located at the edge of the neural fold go through an epithelial-to-mesenchymal transition and delaminate from the dorsal neural tube. Multipotent NCCs then migrate through highly conserved pathways and give rise to a variety of cell types, including skeletal tissues, pigment cells, and neurons and glia of the peripheral nervous system (PNS) (Mayor and Theveneau, 2013). Given that NCCs are highly migratory and colonize the entire developing embryo before the infiltration of professional phagocytes, we hypothesized that they may have the ability to clear cellular debris at early developmental stages.
To examine whether NCCs are capable of clearing debris, we performed in vivo, time-lapse imaging in transgenic embryos expressing fluorescent proteins in NCCs. Excitingly, in these movies, we observed that some fluorescently-labeled NCCs migrated towards dead cells located far away from their innate migratory pathways. Then, they reached toward dead cells and engulfed them, resulting in the formation of large engulfment vesicles inside NCCs.
To confirm that the NCC engulfment process was similar to phagocytosis in professional phagocytes, we imaged transgenic lines labeling early endosomes (PI(3)P sensor) and lysosomes (Lamp1-GFP) (Rasmussen et al., 2015). In these movies, we found that, similar to the maturation of phagosomes, NCC engulfment vesicles fused with early endosomes and lysosomes, leading to progressive acidification inside the vacuoles. Interestingly, we observed NCCs phagocytosing a variety of dead cells, including dead NCCs, neuronal debris, and muscle cells.
In these movies, we also observed that PNS NCCs migrated through motor exit point transition zones, where spinal motor neurons send their axons into the PNS, into the ventral neural tube, and phagocytosed CNS debris. Most of these CNS-located NCCs stayed inside the neural tube for 2 to 12 hours and returned to the PNS through motor exit point transition zones. To test whether PNS NCCs were recruited into the spinal cord by cellular debris, we induced CNS cell death by ablating radial glia using nitroreductase-mediated cell death (Smith et al., 2014; Johnson et al., 2016) and observed a significant increase in the number NCCs recruited into the CNS compared to control embryos. Therefore, we conclude that NCCs can migrate toward and phagocytose dead cells in both CNS and PNS.
Are There Subpopulations of Neural Crest Cells?
Interestingly, we noticed dead cells were not always cleared by the nearest NCC. Instead, under most circumstances, they were phagocytosed by NCCs that came from a distance. And that led us to wonder if phagocytic NCCs were a specific subgroup of the neural crest population. To examine whether phagocytic NCCs belonged to a specific lineage, we performed lineage tracing on phagocytic NCCs expressing a photoconvertible protein. Contrary to our expectations, we observed phagocytic NCCs differentiate into a variety of derivatives, including pigment cells, motor axon-associated cells, and dorsal root ganglia cells, suggesting that phagocytic NCCs are not lineage restricted.
Additionally, under physiological conditions, we only observed 5-10% of NCCs that were phagocytic. To examine whether more NCCs could be “activated”, we induced cell death using laser ablation. Surprisingly, immediately after the ablation, the majority of neighboring NCCs started to engulf cellular debris, resulting in the formation of massive numbers of phagocytic vesicles. These results demonstrated that not only could NCC phagocytic abilities be induced by acute cell death, but also that the majority of NCCs have the potential to phagocytose debris. Therefore, these data are consistent with the hypothesis that phagocytic NCCs are not a specialized subpopulation of the neural crest population.
Phagocytic Neural Crest Cells Break Migratory Rules
Previous studies show that NCCs migrate along conserved, segmentally restricted pathways. However, we found that phagocytic NCCs move toward cellular debris and sometimes even crossed somite boundaries. So what is the mechanism that directs phagocytic NCCs toward dead cells? To answer this question, we designed an ablation assay to quantify NCC recruitment and inhibited a variety of signaling pathways. We found that NCC recruitment was compromised when we treated embryos with a Caspase-1 inhibitor or a interleukin (IL)-1 receptor antagonist, indicating NCC recruitment was mediated by the IL-1β signaling pathway. Interestingly, we observed IL-1β expression in both cellular debris and phagocytic NCCs. Moreover, the IL-1β level we observed after cell ablation was significantly lower than that in previous studies using spinal cord transection and bacterial infection (Bernut et al., 2014; Nguyen-Chi et al., 2014). Given that our cell ablation is more precise compared to manipulations performed in previous studies, the low level of Il-1β release supports the hypothesis that Il-1β secretion is tightly regulated and dependent upon the strength of the inflammatory stimulus (Lopez-Castejon and Brough, 2011).
Conclusion (Yunlu Zhu)
I joined the Kucenas lab at the University of Virginia in early 2014 because I was astonished by the beauty of live imaging in zebrafish embryos (Figure 2).
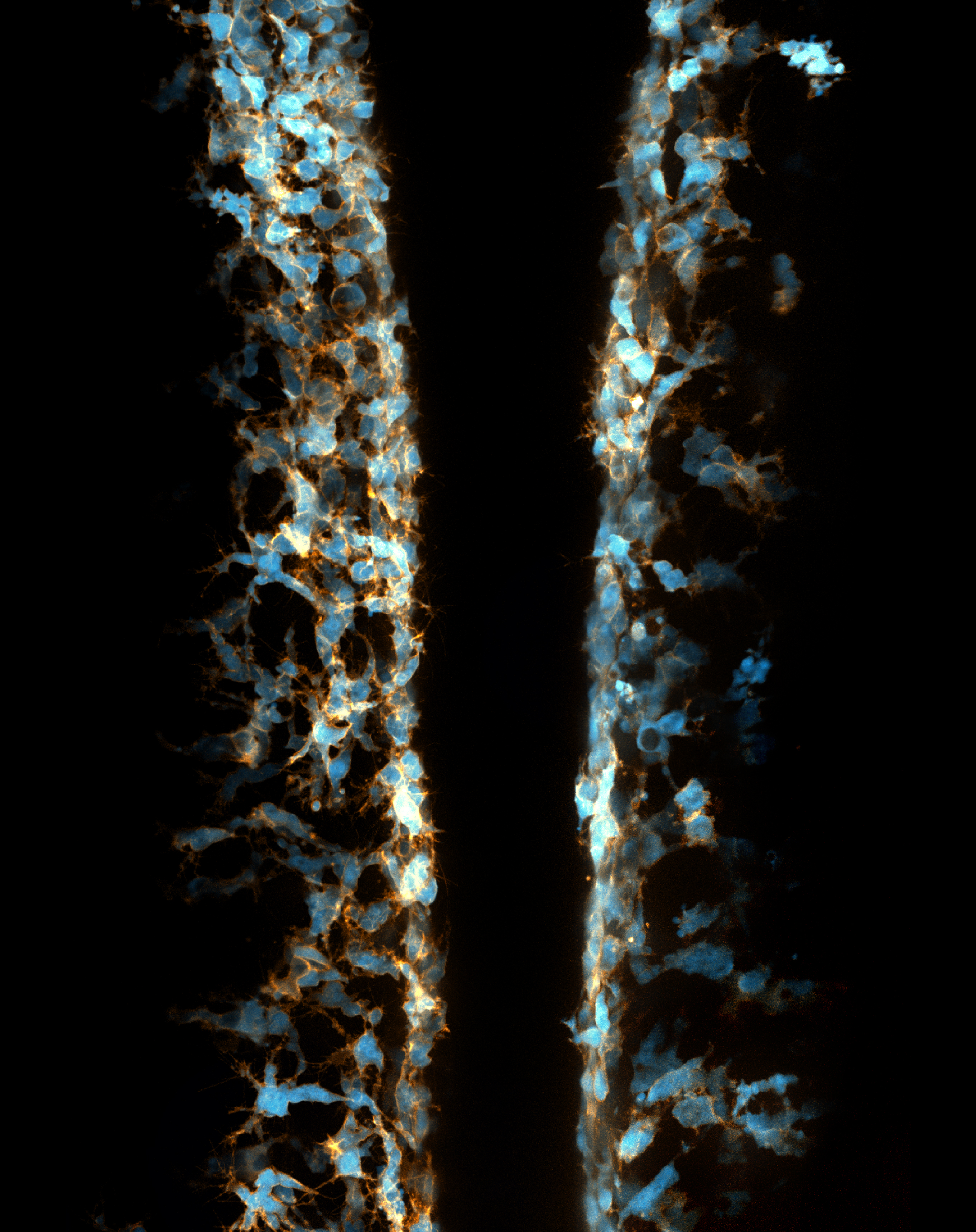
My previous focus was the development of perineurial glia and the role of cell-cell interactions in the development of spinal motor nerves. However, while imaging transgenic embryos labeling NCCs, I accidentally observed that some NCCs migrated ectopically away from their innate pathways and had spherical vacuoles with diameters of 3 to 10 µm. I discovered that these weird behaviors of NCCs had never before been described and was deeply attracted by this phenomenon. Therefore, I shifted my focus toward this NCC project.
This novel role of NCCs in debris clearance was quite unexpected but perfectly reasonable. However, the nature of these phagocytic NCCs is not well understood and we have many remaining questions. Given that the majority of NCCs are capable of phagocytosing debris, why don’t they respond to cell death under physiological conditions? What is special about those active NCCs that migrate towards dead cells from a distance? And what happens when professional phagocytes like macrophages colonize the trunk region of the developing embryo? Are their immune-NCC interactions? And what happens if one of these two populations is unable to clear debris? There are so many new questions that have come from this work, and I’m excited to see which ones the next students decide to pursue.
I would like to end this post with a quote from Yogi Berra, which, I think, precisely describes the beauty of live imaging and my experience in this project: “You can observe a lot by just watching”.
References:
Arya, R. and White, K. (2015) ‘Cell death in development: Signaling pathways and core mechanisms’, Seminars in Cell & Developmental Biology. Academic Press, 39, pp. 12–19. doi: 10.1016/J.SEMCDB.2015.02.001.
Bernut, A. et al. (2014) ‘Mycobacterium abscessus cording prevents phagocytosis and promotes abscess formation.’, Proceedings of the National Academy of Sciences of the United States of America. National Academy of Sciences, 111(10), pp. E943-52. doi: 10.1073/pnas.1321390111.
Bertrand, J. Y. et al. (2013) ‘Three pathways to mature macrophages in the early mouse yolk sac Three pathways to mature macrophages in the early mouse yolk sac’, Blood, 106(9), pp. 3004–3011. doi: 10.1182/blood-2005-02-0461.
Galluzzi, L. et al. (2018) ‘Molecular mechanisms of cell death: recommendations of the Nomenclature Committee on Cell Death 2018’, Cell Death & Differentiation. Nature Publishing Group, 25(3), pp. 486–541. doi: 10.1038/s41418-017-0012-4.
Herbomel, P., Thisse, B. and Thisse, C. (1999) ‘Ontogeny and behaviour of early macrophages in the zebrafish embryo.’, Development (Cambridge, England), 126(17), pp. 3735–45.
Herbomel, P., Thisse, B. and Thisse, C. (2001) ‘Zebrafish early macrophages colonize cephalic mesenchyme and developing brain, retina, and epidermis through a M-CSF receptor-dependent invasive process.’, Developmental biology, 238(2), pp. 274–88. doi: 10.1006/dbio.2001.0393.
Johnson, K. et al. (2016) ‘Gfap-positive radial glial cells are an essential progenitor population for later-born neurons and glia in the zebrafish spinal cord’, Glia, 64(7), pp. 1170–1189. doi: 10.1002/glia.22990.
Lopez-Castejon, G. and Brough, D. (2011) ‘Understanding the mechanism of IL-1β secretion’, Cytokine and Growth Factor Reviews. Elsevier Ltd, 22(4), pp. 189–195. doi: 10.1016/j.cytogfr.2011.10.001.
Mayhew, T. M. et al. (1999) ‘Epithelial integrity, cell death and cell loss in mammalian small intestine.’, Histology and histopathology, 14(1), pp. 257–67. doi: 10.14670/HH-14.257.
Mayor, R. and Theveneau, E. (2013) ‘The neural crest’, Development, 140(11), pp. 2247–2251. doi: 10.1242/dev.091751.
McGrath, K. E. et al. (2003) ‘Circulation is established in a stepwise pattern in the mammalian embryo.’, Blood. American Society of Hematology, 101(5), pp. 1669–76. doi: 10.1182/blood-2002-08-2531.
Nguyen-Chi, M. et al. (2014) ‘Transient infection of the zebrafish notochord with E. coli induces chronic inflammation’, Disease Models & Mechanisms, 7(7), pp. 871–882. doi: 10.1242/dmm.014498.
Rasmussen, J. P. et al. (2015) ‘Vertebrate epidermal cells are broad-specificity phagocytes that clear sensory axon debris.’, The Journal of neuroscience : the official journal of the Society for Neuroscience. Society for Neuroscience, 35(2), pp. 559–70. doi: 10.1523/JNEUROSCI.3613-14.2015.
Smith, C. J. et al. (2014) ‘Contact-Mediated Inhibition Between Oligodendrocyte Progenitor Cells and Motor Exit Point Glia Establishes the Spinal Cord Transition Zone’, PLoS Biology. Edited by B. A. Barres, 12(9), p. e1001961. doi: 10.1371/journal.pbio.1001961.
Stremmel, C. et al. (2018) ‘Yolk sac macrophage progenitors traffic to the embryo during defined stages of development’, Nature Communications. Nature Publishing Group, 9(1), p. 75. doi: 10.1038/s41467-017-02492-2.
Zhu, Y. et al. (2019) ‘Migratory Neural Crest Cells Phagocytose Dead Cells in the Developing Nervous System’, Cell, 179(1), pp. 74-89.e10. doi: 10.1016/j.cell.2019.08.001.