Embryonic hydraulics triumphs
Posted by Chii J Chan, on 26 June 2019
In this article I share with you a more personal, chronological account of how our story unfolds (recently published in Nature), and highlight some key events and insights that help guide the direction of the study, which are not described in the publication. Readers are welcome to refer to the publication for more technical details.
Quantifying blastocoel pressure in vivo
The defining feature of mammalian development is the formation of a blastocyst with a fluid-filled cavity, termed blastocoel. However, the physiological function of blastocoel in mammalian blastocysts, and the influence of fluid pressure on tissue mechanics, embryos size and fate specification remain largely unknown. Trained as an experimentalist in cell mechanics, my first thought when I conceived the project was to devise a method to characterize the spatiotemporal change in the blastocoel pressure during mouse blastocyst development. Surprisingly very few studies have quantified lumen pressure in embryonic development. Precise quantification of the blastocoel pressure will allow us to address if the pressure grows or decreases with blastocyst expansion (the latter case analogous to a balloon, where its internal pressure drops as it inflates, which is why it is easier to blow up a large balloon). Initially we tried to characterize the pressure indirectly by using the Laplace law, where pressure is equal to the tissue surface tension divided by the cavity size. However we found that the measured pressure scales with the pipette size, which cannot be the case. This leads us to explore alternative methods to directly quantify the lumen pressure. Inspired by the works of Ryan Petrie, who used a micropressure probe to measure intracellular pressure1, we wondered whether we could apply a similar technique to mouse blastocysts. Bringing with me some mouse blastocysts and starfish oocytes (as a control, thanks to Johanna Bischof!), I then made a daring trip to Ryan’s lab at Drexel University in Pennsylvania to test the feasibility of the device in measuring blastocoel pressure (Fig. 1a). I still remember how thrilled we were when we made our first successful measurement (Fig. 1b), which was found to be surprisingly high (~1500 Pa), certainly higher than the known cytoplasmic pressure2. Overall it proved to be an extremely fruitful trip, all thanks to Ryan’s generosity and patience. Here I would also like to acknowledge the assistance of A.K. Hadjantonakis for providing us with ‘back-up’ mice during the trip.
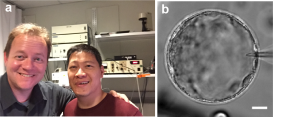
Blastocyst collapse is triggered by mitosis rather than random rupture of cell-cell junctions
While we found that the pressure and the trophectoderm (TE) cortical tension grow with blastocyst maturation, we also observed that the blastocysts exhibit regular patterns of collapse followed by re-expansion. Surprisingly few studies have tackled what precisely drives this dynamics, a topic I proposed to study in my first year lab retreat in the Hiiragi lab (I called it ‘lumen breathing’, a term that raised many eyebrows in the lab!). My natural hypothesis, similar to what many in the field have speculated, is that these events are likely stochastic and are driven by random rupture of TE cell-cell junctions, when the cell tension exceeds the threshold cell-cell adhesion strength. By this time we had already observed that the TE cells in the reduced embryos (e.g. quarter embryo is derived from a single blastomere from a four-cell stage embryos) have a higher cortical tension than that of the full embryos at early blastocyst stage, so the reduced embryos should show more frequent collapse events, which proves to be the case! While this shows clearly that higher cortical tension leads to more frequent collapse dynamics, it still does not answer what ‘triggers’ the collapse. Interestingly, we found that blastocyst collapse is not triggered by some random events of junctional rupture. Instead they are triggered by mitosis of mural TE cells, which we carefully confirmed with a series of pharmacological and embryological perturbations. This experience taught me to always check my assumptions carefully – Nature always has new surprises for us!
Mechanosensing is crucial for blastocyst development
While it provides great satisfaction for me to understand how the blastocyst regulates its size, we wondered if lumen size control has important functions in blastocyst development (which is one thing I learned in a biological lab: to ask not just how, but also why). One question we asked was whether the growth in lumenal pressure impacts the development of TE cell-cell junctions, which are crucial for tissue integrity (adherens junctions) and blastocyst sealing (tight junctions). Intuitively we thought that relaxing cell contractility and TE tension should lead to a larger cavity (as observed in epithelial dome3), but to our surprise we found a smaller cavity when we abolished actomyosin contractility. This suggests that there could be an active response from the TE epithelium that ensures proper lumen expansion. Indeed we found that increased cortical tension leads to an active recruitment of junctional proteins (such as vinculin, Fig. 2a) that seals the tight junctions, which possibly functions to counterbalance the higher pressure building up during blastocyst maturation. This is a beautiful example of positive feedback control that exists so abundantly in developmental processes. However, to confirm this, we still needed to observe these events directly. Here we struggled for a few months. We tried loading fluorescently labelled dextran into the blastocoel and tracing the leakage sites, which proved futile since the very weak signal at the leakage site was ‘drowned’ out by the strong background signal in the blastocoel. Finally, during a joint retreat with Carl-Philipp Heisenberg’s lab, I learnt of a cool technique to monitor junctional leakage based on Fluozinc leakage (thanks to C. Schwayer, T. Higashi and A. Miller, whose work was recently published4). This method finally gave us a good signal-to-noise ratio to unambiguously confirm leakage due to transient reduction of cortical tension (Fig. 2b).
One question that arose early on in our study (also commented by a keen reviewer) is if cell stretching caused by lumen expansion promotes cell division in TE cells, as observed in other epithelia5. To our disappointment, we found no difference in the cell cycle length between the more-stretched TE cells in late blastocysts and those of the less-stretched ones in early blastocysts, which suggest that stretch-induced cell division may not play a role in mouse preimplantation development.
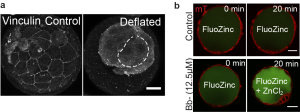
Towards a theoretical model
At this point we approached Teresa Ruiz-Herrero and L. Mahadevan from Havard to see if we can build a theoretical model that recapitulates our experimental findings. Building on their previous model6, it did not take long before they came up with the simulations that could explain the key features of our experiments. However the true merit of a model is to make predictions that could be tested experimentally. A key prediction of the model is that the steady-state blastocyst size is governed by the ratio of tissue yield stress and tissue elasticity, as well as the initial tissue size. To verify this prediction, we embryologically manipulated the initial tissue size by making reduced and amplified blastocysts. Knowing the tissue yield stress from the measured blastocoel pressure and tissue thickness, we were able to extract the tissue elasticity. With the help of Martin Bergert and Alba Diz-Muñoz at EMBL, we were able to use the atomic force microscopy to measure the tissue elasticity of TE, which nicely falls within the range predicted by the model.
Lumen size impacts tissue architecture and cell fate
Early on in the project we postulated that another function of lumen may be its regulation of tissue architecture and cell fate specification. After all, the lumen takes a significant portion of the entire embryo, and its presence must impact how the cells are allocated to the inside versus the outside of the blastocyst. In fact it is not difficult to think that given the same tissue volume (cell number x cell volume), a blastocyst with a smaller cavity would have more cells allocated to the inner layer, compared to one with a larger cavity, purely by geometric argument. This means that the ICM-to-TE cell ratio should be higher in blastocysts with a reduced cavity size. This is indeed what we see with both pharmacological and genetic perturbation studies (Fig. 3a). Still, it remained unclear what is the cellular mechanism driving this change in ICM/TE ratio. We then considered three scenarios: a). less ‘asymmetric’ division of inner cells that give rise to daughter cells that end up on the outer layer, b). more asymmetric division of outer TE cells to generate an inner daughter cell, and c). active cell migration from the outside (polar TE) to the inside (ICM). While discussing with Dimitri Fabrigès, an expert in lightsheet microscopy within the lab, I learnt that scenario a) and c) are almost never observed during blastocyst expansion phase. I therefore focused on investigating scenario b). At this point I recalled some works in the past where people were able to track the TE lineage in preimplantation stage7, using a DII dye that stays with the outer cells through subsequent generations. Furthermore, combining this with live marker CDX2-GFP x H2B-mCherry, I was able to reliably track the movement and final position of the inside and the outside cells. Using this approach, we indeed observed more DII-labelled cells in the ICM of blastocyst with reduced cavity size, than those in the WT. Importantly, we also found that the internalized cells, following asymmetric TE division, eventually lose their CDX2 signal and adopt an inner cell fate, consistent with our hypothesis based on scenario b) (Fig. 3b). Our results are consistent with some classic papers by Rossant et. al. showing that TE and ICM cell fates remain plastic during the blastocyst expansion phase (16-32 cell stage)8,9. In my view, this is a beautiful finding because it reveals that lumen pressure can influence the division pattern of cells around it, and thereby lead to changes in cell position and eventual fate specification (through the HIPPO pathway). While it is known that cell shape and force can guide spindle orientation10, this is the first time we observe that the lumen pressure could directly influence the division pattern of cells in vivo and impact their cell fate specification.
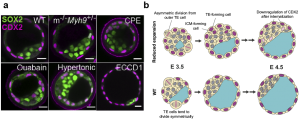
Outlook and open questions
This work opens up several new directions, even beyond mouse mechanobiology. First, in terms of technique, the micropressure probe allows us to directly measure hydrostatic pressure in vivo, independent of the geometry of the sample, which is often required for indirect determination of pressure (e.g. round cells as in Laplace’s Law). Furthermore this device allows us to quantify spatial difference in pressure within a tissue (for example, we measured a higher pressure in the nucleus compared to the cytoplasmic pressure in starfish oocytes). In the future it will be exciting to investigate the role of fluid pressure in organ development, such as in lungs and kidneys, and in other model systems such as sea animals (body extension) and organoids (symmetry breaking). Second, I think this study really highlights a critical role of lumen pressure in tissue mechanics (apart from the conventional key players such as actomyosin contractility or cell-cell adhesion). In the future it will be interesting to investigate if lumenal pressure plays a similar role in guiding cell division and cell positioning in other developmental processes.
Are there other evidence that lumen pressure may trigger mechanosensing or mechanotransduction in mouse blastocysts? We could hypothesize a few. For example, does cell stretching due to lumen expansion lead to YAP signaling in the TE cells, which then act to stabilize its CDX2 expression? Also, it is known that mural TE cells eventually ‘invert’ their polarity as it enters the peri-implantation stage, where the lumen-facing side becomes apical and the outer surface becomes basal. It is believed that this facilitates the mural TE cells to adhere to the surrounding tissue as the blastocyst embeds itself into the uterine wall. Does luminal pressure play a role here?
One exciting aspect is if lumenal expansion also plays a role in the second lineage segregation during the mid-to late blastocyst stage, where the ICM further segregates into the epiblast and the primitive endoderm11. Indeed, using a combination of pharmacological and biophysical approach, work in our lab has shown that lumen expansion is required for proper EPI-PrE cell fate specification, as well as their spatial segregation12, suggesting that the lumen may provide mechanical and biochemical cues (pressure, contact-free surface, signalling niche) to impact cell fate specification. This provides further evidence that lumen morphogenesis is an integral part of tissue patterning in mammalian preimplantation development.
I feel extremely privileged to have the opportunity to work with many great scientists along this incredible journey. These experiences truly enriched me on both the professional and personal level.It also taught me the benefits of engaging in an interdisciplinary approach early on in the project, which also means taking risks, get out of your comfort zone, and approaching people with the right expertise to learn new techniques and concepts. In the long run this also allows one to build a strong network with fellow scientists, and many will go on to become close friends, as in my case.
References
- Petrie, R. J., Koo, H. & Yamada, K. M. Generation of compartmentalized pressure by a nuclear piston governs cell motility in a 3D matrix. Science 345, 1062–1065 (2014).
- Stewart, M. P. et al.Hydrostatic pressure and the actomyosin cortex drive mitotic cell rounding. Nature 469, 226–231 (2011).
- Latorre, E., Kale, S., Casares, L., Gomez-gonzalez, M. & Uroz, M. Active superelasticity in three-dimensional epithelia of controlled shape. Nature 563, 203–208 (2018).
- Stephenson, R. E. et al.Rho flares repair local tight junction leaks. Dev. Cell 48, 445–459 (2019).
- Gudipaty, S. A. et al.Mechanical stretch triggers rapid epithelial cell division through Piezo1. Nature1–12 (2017).
- Ruiz-Herrero, T., Alessandri, K., Gurchenkov, B. V., Nassoy, P. & Mahadevan, L. Organ size control via hydraulically gated oscillations.Development 144, 4422–4427 (2017).
- Krupa, M. et al.Allocation of inner cells to epiblast vs primitive endoderm in the mouse embryo is biased but not determined by the round of asymmetric divisions (8-16- and 16-32-cells). Dev. Biol. 385, 136–148 (2014).
- Rossant, J. & Lis, W. T. Potential of isolated mouse inner cell masses to form trophectoderm derivatives in vivo. Dev. Biol. 70, 255–261 (1979).
- Rossant, J. & Vijh, K. M. Ability of outside cells from preimplantation mouse embryos to form inner cell mass derivatives. Dev. Biol. 482, 475–482 (1980).
- Xiong, F. et al.Interplay of cell shape and division orientation promotes robust morphogenesis of developing epithelia. Cell 159, 415–427 (2014).
- Chazaud, C. & Yamanaka, Y. Lineage specification in the mouse preimplantation embryo. Development 143, 1063–1074 (2016).
- Ryan, A. Q., Chan, C. J., Graner, F. & Hiiragi, T. Lumen expansion facilitates epiblast-primitive endoderm fate specification in the mouse blastocyst formation. BioRxiv https://doi.org/10.1101/575282