High-speed imaging of entire organisms at sub-cellular, isotropic resolution
Posted by Raghav Chhetri, Janelia Research Campus, on 14 December 2015
A microscope has long remained a biologist’s favorite tool, and for obvious reasons, as it has been the tool to continually grant us deeper access into the elusive world that has always remained close and yet, typically, out of sight. Recent advancements in light sources, detectors, opto-mechanical components, and powerful computing frameworks have collectively accelerated the field of bio-imaging. So, what once was a qualitative and curious glance into the microscopic world beneath us is now a quantitative field of imaging the micro- and the nano- world.
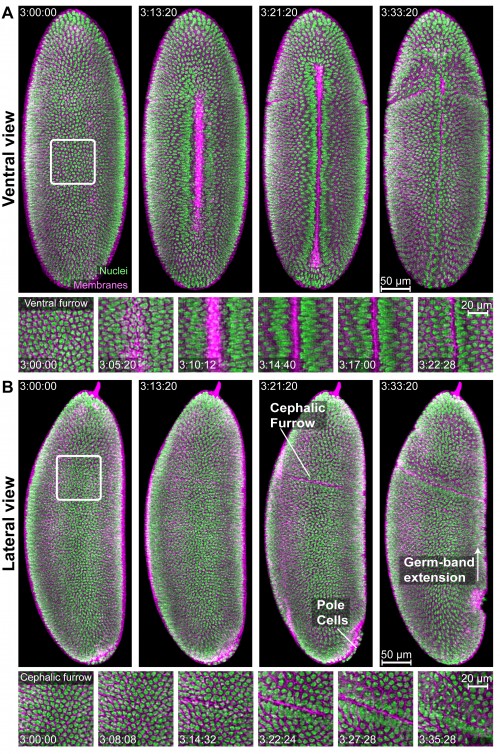
More recently, recording live specimens in three-dimension over an extended period of time has been made possible with the application of light-sheet microscopy in imaging fluorescently-tagged biological specimens [1,2]. Despite affording the flexibility to gently assess a live specimen with reduced photobleaching and phototoxicity, a majority of high-end light-sheet microscopes available today still suffer from anisotropy in spatial resolution, in much the same way as a wide-field microscope. The resolution along the direction sampled by the objective for depth-sectioning tends to be much worse than that in the plane viewed by the objective. In other words, the axial (z- or depth) resolution is much worse than the lateral (x- and y-) resolution. This glaring anisotropy in spatial resolution stems from the way an objective collects the emitted photons from a fluorescent object. Although a fluorescent object emits photons in all directions, a detection objective only captures photons that are emitted within a cone of its viewing angle (defined also by a parameter called the numerical aperture or NA). Even high-NA lenses in immersion media fail to capture the entirety of the photons emitted by the sample, and as such, much less information is gathered about the axial content of the object compared to the lateral content. Thus, the anisotropy in the spatial resolution isn’t an artifact of under-sampling in the axial direction, but rather a physical limitation imposed by the very way an objective collects the emitted photons.
In light-sheet microscopy, the anisotropy in spatial resolution can be mitigated by either making the light-sheet quite thin as in a lattice light-sheet microscope [3] or collecting the image data along multiple viewing angles [4,5]. The first approach however limits the overall coverage of the sample we can image and also takes longer to scan across a relatively large sample. In the context of imaging large specimens at a high temporal resolution, this approach thus tends to be limiting. The second approach of acquiring volume stacks from multiple viewing angles does indeed offer a solution to the problem of resolution anisotropy, but the implementation has either been through the rotation of the sample at various angles to acquire multi-view image-stacks [4] or, more recently, via a pair of shared illumination and detection objectives as in the dual-view plane illumination microscope (diSPIM) [5]. Rotating the sample in multiple orientations for the acquisition of multi-view image-stacks penalizes the overall acquisition time, and thus limits imaging to processes that don’t have a stringent temporal sampling requirement. Using a pair of shared illumination and detection objectives to sequentially capture the image-stacks along two orthogonal directions, as in the now commercial diSPIM microscope, offers a user-friendly implementation for use in a laboratory workbench, but this method is limited to small, transparent samples such as C. elegans embryos.
Today, the frontiers of live three-dimensional imaging has also reached the domain of recording neuronal activities across the whole-brain of a live animal [6,7], which places high demands on the spatio-temporal resolution to reliably discern neuronal activities from within an ensemble of densely packed neuronal population. To make accurate predictions of the temporal and spatial dynamics of such processes, there has indeed been an immense need for an imaging tool that not only captures three-dimensional images at an isotropic, sub-cellular resolution, but also does so at a high temporal resolution and over an extended period of time in large and non-transparent animal models such as Drosophila and zebrafish, for which a plethora of genetic tools are already available and are constantly being updated.
The development of isotropic multi-view (IsoView) light-sheet microscopy overcomes the spatio-temporal resolution and physical coverage limitations of earlier approaches and now allows isotropic, sub-cellular resolution imaging of large, non-transparent samples at a high temporal resolution [8]. The IsoView design utilizes an orthogonal arrangement of four shared illumination and detection objectives surrounding a sample. Thus, the axial dimension along two opposing views becomes the lateral dimension along the orthogonal views, and the fusion of the image content from all four views and the subsequent deconvolution of the multi-view images results in a spatially isotropic three-dimensional representation of the sample. Additionally, IsoView enables simultaneous four-view imaging by spatially offsetting the orthogonal light-sheet scans in the vertical direction and equally offsetting the active row of pixels in the orthogonal cameras. As such, although all four light-sheets and all four cameras are operated simultaneously, neither the illumination beams in the orthogonal illumination arms nor the active row of camera pixels in the orthogonal detection arms cross paths with one another. In this manner, the simultaneous acquisition of four views doesn’t sacrifice the volumetric acquisition speed for spatially isotropic imaging and allows one to follow fast, dynamic processes with sub-second temporal resolution. In characterizing the IsoView system performance, we measured the isotropic resolution to be approximately 450 nm using fluorescent beads embedded in agarose. Furthermore, we measured the effective isotropic resolution to be 1-2 microns in vivo by characterizing fluorescent beads injected at various depths in a Drosophila embryo, which better mimics the actual performance in an optically challenging biological environment.
As our first demonstration, we performed IsoView imaging of an entire 1st instar Drosophila larva expressing cytoplasmic GCaMP throughout its nervous system at a rate of 2 volumes/sec over a period of over 8 hours— an illustration of a functional imaging experiment performed at developmental timescales. To the best of our knowledge, this is the first demonstration of whole-animal functional imaging performed in a higher invertebrate. The IsoView images from this recording show cellular and sub-cellular morphologies with unprecedented details along all viewing angles, thus constituting an accurate three-dimensional representation of the sample that no longer suffers from spatial resolution bias along any preferred direction. We also demonstrated isotropic, sub-cellular resolution functional imaging at a sustained rate of 1 volume/sec in a 3-day old zebrafish larva expressing GCaMP throughout its nervous system. To the best of our knowledge, this constitutes a first demonstration of spatially isotropic, sub-cellular functional imaging of a whole-brain in a vertebrate. The images from this recording show the power afforded by IsoView in unambiguously discerning single neurons even from within a dense ensemble of neurons located deep inside a large specimen such as a zebrafish larva, the size of which is approximately 400-fold larger than C. elegans embryos. Lastly, a demonstration of simultaneous two-color imaging was also performed in a gastrulating Drosophila embryo expressing nuclear-localized RFP and membrane-localized GFP. The entire volume of the embryo was acquired from four views in both color channels every 4 seconds, which offers good temporal resolution to capture in detail key events during gastrulation, such as ventral furrow formation, cephalic furrow formation, movement of pole cells, and germ-band extension (Figure). Owing to the isotropic micron-level resolution, we were able to reliably distinguish neighboring cells and resolve morphological features at the subcellular level across the entire embryo in the 2-color recording of the gastrulating Drosophila embryo.
The above discussed demonstrations of 2 Hz whole-animal imaging in a Drosophila embryo (and eventually, larva) for over 8 hours, 1 Hz whole-brain imaging in a 3-day old zebrafish larva, and simultaneous two-color imaging of a gastrulating Drosophila embryo show the breadth of imaging IsoView microscopy allows. Importantly, IsoView affords us the ability to perform truly three-dimensional analysis of fast cellular dynamics and neuronal activities across an entire animal and yet preserve sub-cellular resolution and temporal precision to key in on individual cells locally. With the combination of high temporal resolution, high spatial resolution, and large physical coverage, we anticipate that IsoView imaging will open doors to an array of live-imaging applications ranging from developmental systems biology to systems neuroscience.
Explore further:
IsoView video: Whole-animal functional imaging of a Drosophila embryo
IsoView video: Long-term, high-speed timelapse IsoView recording of Drosophila embryo development
Fore more information:
References:
1. Huisken, J., Swoger, J., Del Bene, F., Wittbrodt, J. & Stelzer, E. H. K. Optical sectioning deep inside live embryos by selective plane illumination microscopy. Science 305, 1007–9 (2004).
2. Keller, P. J., Schmidt, A. D., Wittbrodt, J. & Stelzer, E. H. K. Reconstruction of zebrafish early embryonic development by scanned light sheet microscopy. Science 322, 1065–9 (2008).
3. Chen, B.-C. et al. Lattice light-sheet microscopy: Imaging molecules to embryos at high spatiotemporal resolution. Science 346, 1257998 (2014).
4. Swoger, J., Verveer, P., Greger, K., Huisken, J. & Stelzer, E. H. K. Multi-view image fusion improves resolution in three-dimensional microscopy. Opt. Express 15, 8029–42 (2007).
5. Wu, Y. et al. Spatially isotropic four-dimensional imaging with dual-view plane illumination microscopy. Nat. Biotechnol. (2013).
6. Ahrens, M. B., Orger, M. B., Robson, D. N., Li, J. M. & Keller, P. J. Whole-brain functional imaging at cellular resolution using light-sheet microscopy. Nat Methods 10, 413–420 (2013).
7. Lemon, W. C. et al. Whole-central nervous system functional imaging in larval Drosophila. Nat. Commun. 6, 7924 (2015).
8. Chhetri, R. K. et al. Whole-animal functional and developmental imaging with isotropic spatial resolution. Nat. Methods 12, 1171–78 (2015).