Making a map: exploring the origins of the shoulder and neck
Posted by Shunya Kuroda, on 18 September 2024
Maps are vital tools for any exploration, guiding us through the unknown and helping us make sense of the world. For some developmental biologists, a map refers to a fate map. During embryonic development, a single fertilized egg divides and differentiates into various cell types. Thus, determining cell lineages over time and space in small embryos is a critical step in understanding how our body is built. One of the earliest experimental embryologists, Vogt’s seminal work with ‘vital dye’ to trace cell lineages and migration paths during amphibian embryo gastrulation remains valuable even today, despite the technological limitations of his time [Vogt, 1929]. With the more sophisticated techniques we have now, what kind of maps might we be able to create?
In our recent paper [Kuroda et al., 2024], we combined modern techniques to elucidate the complex developmental origins of the pectoral girdle in zebrafish, labeling the cells that potentially contribute to the pectoral girdle from the early developmental stage long before the formation of skeletons. By comparing this cell fate map of the fish pectoral girdle with the preexisting map of the amniote shoulder girdle, we were able to infer how the shoulder/pectoral skeletons were rearranged during the transition of vertebrates from water to land. Below, I would like to share the background scene of our research.
How I started this project
After getting my Ph.D. degree in Japan, I was fortunate enough to be hired as a postdoc in the Tetsuya Nakamura lab. Tetsuya has been researching the key morphological evolution involved in the water-to-land transition, mainly from embryological and genomic perspectives. After revealing the hidden and surprising commonality of developmental origins between fins and hands [Nakamura et al., 2016], he established his own lab at Rutgers University in the U.S. The Nakamura lab was also expanding its research scope to the skeletal structure that supports the pectoral appendages, specifically the pectoral girdle, when I moved to his lab.
The ancestry of our shoulder skeleton seems to have already existed in the pectoral girdles of the fish-like body plan before the vertebrate transition from water to land. Phylogenetic evidence for this assumption can be observed in the presence of the scapula and clavicle in extinct lobe-finned fish, such as Eusthenopteron and in extant lobe-finned fish, such as lungfish and coelacanth [Jarvik, 1980]. Their pectoral skeletons had been transformed over generations of bony vertebrates, eventually helping them adapt to terrestrial life by supporting their increased body weight after the transition to land [Romer & Parsons, 1986].
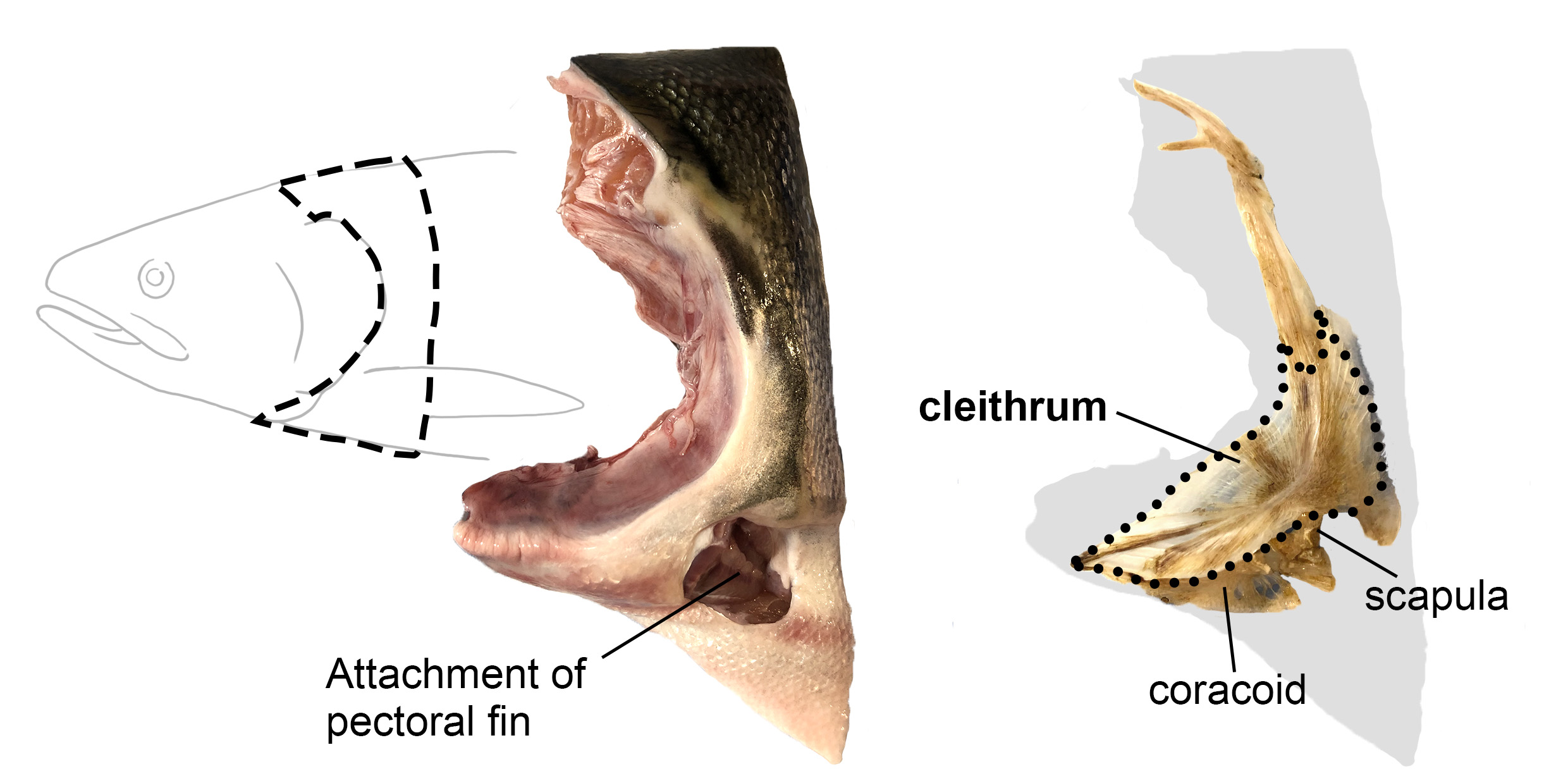
Although the scapula and clavicle were cherished and used over long geological periods, some bones were reduced or completely lost during and/or after the water-to-land transition. Among them, the cleithrum is one of the most mysterious bones. Originally, the cleithrum in aquatic fish was referred to as the clavicle. However, Gegenbaur discovered the true clavicle in fish and thus renamed the bone originally called the clavicle as the cleithrum [Gegenbaur, 1895]. The relative size of the cleithrum gradually decreased after the transition to land and disappeared multiple times independently in tetrapod lineages [Gess & Ahlberg, 2018; Matsuoka et al., 2005].
When I joined the Nakamura lab, some members had already created a mutant zebrafish with gli3 gene and discovered that this mutant exhibited an interesting phenotype: an enlarged scapulocoracoid (a cartilaginous precursor for the scapula and coracoid) and cleithrum reduction. It was as if these changes mimicked the pectoral girdle morphological changes that occurred during the water-to-land transition in bony vertebrates! They were beginning to uncover that the ratio of scapulocoracoid to cleithrum changes depending on the magnitude of the Hedgehog-Gli signaling and its downstream pathways [Wei et al., 2023]. To better understand what was happening in this mutant fish and, possibly, the actual evolutionary process, I started my project to determine 1) whether the embryonic sources of the fish scapulocoracoid and cleithrum are shared, and 2) whether there are other cell populations involved, including potential contributions from outside the pectoral fin precursors.
How I chose methods for cell lineage tracing
In animals possessing cleithra, the bone forms at the boundary between the head and trunk. So, I narrowed down the candidate cell populations that could contribute to the cleithrum to four groups: two from head mesenchymal populations (cranial neural crest cells and cardiopharyngeal mesoderm (CPM)) and two from trunk mesenchymal populations (anterior somites and fin-field lateral plate mesoderm (LPM)). Once I refined the candidates, all that remained was to investigate whether each of these cell populations contributes to the cleithrum.
There is no perfect method for cell lineage analysis, and the applicability of a particular method is limited to certain occasions or animal species. Thus, several methods should be combined for consistent and reliable conclusions. Fortunately, many tools for cell lineage analysis have been developed in zebrafish, allowing us to test cell lineages of the pectoral girdle with different methodologies. Initially, I considered tracking cell lineages through tissue transplantation, as has been done in zebrafish and medaka in previous studies [Cole et al., 2011; Shimada et al., 2013]. This involves preparing fluorescently labeled zebrafish and unlabeled zebrafish embryos and surgically exchanging tissues between them at the same embryonic stage. The advantage of this method is that, since the fluorescent protein is encoded in the donor’s genome, the label remains stable and doesn’t dilute with cell division in the host embryo, as is the case with vital dyes. This makes it suitable for long-term tracking, such as investigating the developmental origins of the musculoskeletal system. The downside of this method is that a fine surgical skill is required. Imagine precisely cutting out an approximately 100 mm square region from an almost spherical donor embryo, and transplanting it into the target region in a host embryo! Even worse, this procedure has to be performed at the developmental stage before our four target cell populations mingled with each other, i.e., late at night on the day of fertilization. I soon realized that I couldn’t perform such a precise operation with trembling hands coming from sleep deprivation, so I decided to explore other methods. (I have deep respect for the predecessors who managed to pull off such experiments in the past!)
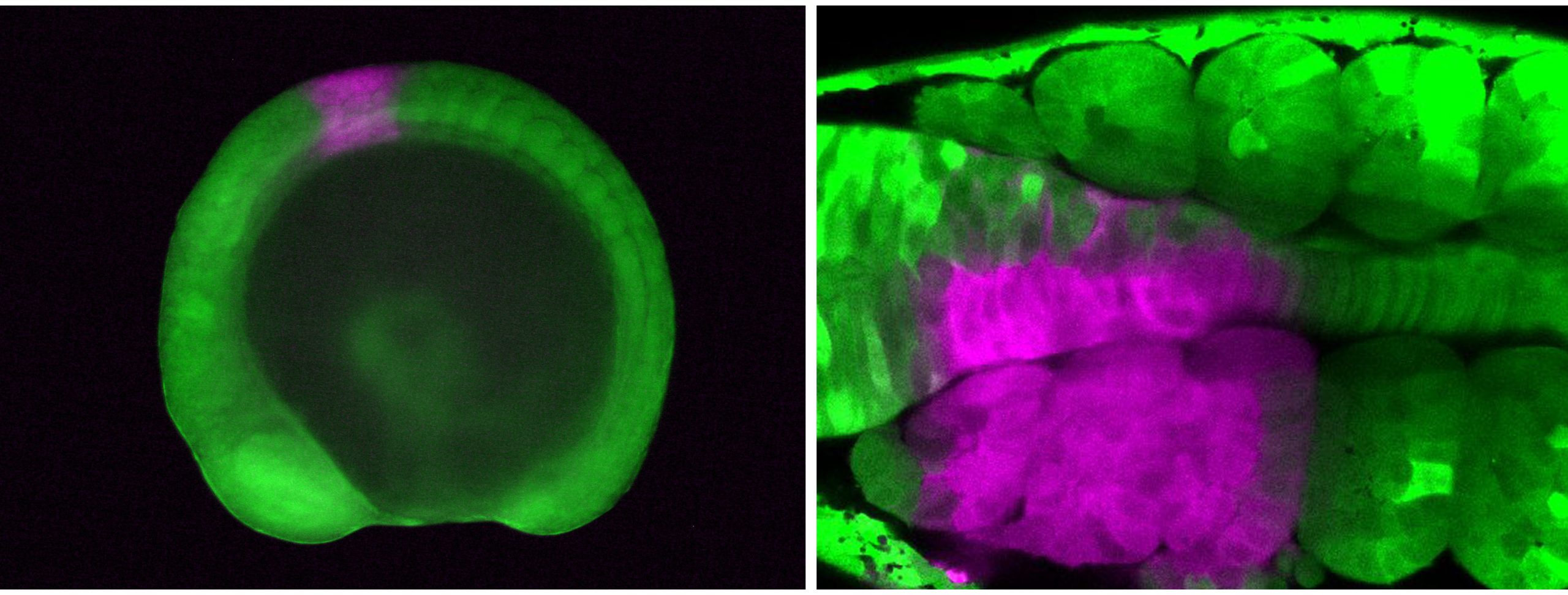
Next, I tried a tracking method using a fluorescent protein called Kaede, which changes color from green to red upon exposure to UV light. I placed zebrafish embryos that express Kaede throughout the body on a confocal microscope stage, determined the target area with the computer monitor, and illuminated the UV laser on the target cells, converting the cell color from green to red. Although working late at night was still necessary, handling the microscope and clicking a computer mouse with trembling hands was much more feasible. This method worked nicely, and I finally succeeded in labeling the cranial neural crest cells, CPM, anterior somites, and fin-field LPM individually!
The downside of manual labeling of the spatially restricted area such as vital dye injection, surgical transplantation, and Kaede-based photoconversion is that the experimental precision depends on the experimenter’s technique. So, we decided to deploy another complementary and more reproducible method, genetic cell lineage analysis. Genetic cell lineage analysis is a technique that utilizes the tissue-specific activity of a cis-regulatory element with an artificial gene induction system, such as Cre/loxp system that permanently labels the target cell population. Of course, the activity of a cis-regulatory element is more or less pleiotropic, so one shouldn’t expect the existence of a perfect ‘marker’ enhancer/promotor. However, genetic cell lineage analysis was still an excellent tool that compensates for the weaknesses of Kaede-based photoconversion. In collaboration with Christian Mosimann and Robert Lalonde at the University of Colorado and Gage Crump and Claire Arata at the University of Southern California, we were able to collect transgenic (Tg) fishes to track progeny cells that experience the following ‘marker’ gene expressions: sox10 and crestin (as markers for neural crest cells), tbx1 (for CPM), draculin (for LPM), and tbx6 (for paraxial mesoderm including anterior somites).
Multiple lineages converge to form a single bone
By combining manual cell labeling by Kaede photoconversion and genetic cell lineage analyses by several transgenic fishes, we eventually found that the cranial neural crest cells, CPM, anterior somites, and fin-field LPM all converge to form the cleithrum. In other words, the cell populations that form the boundary between the head and trunk in vertebrate embryos all converge into the cleithrum.
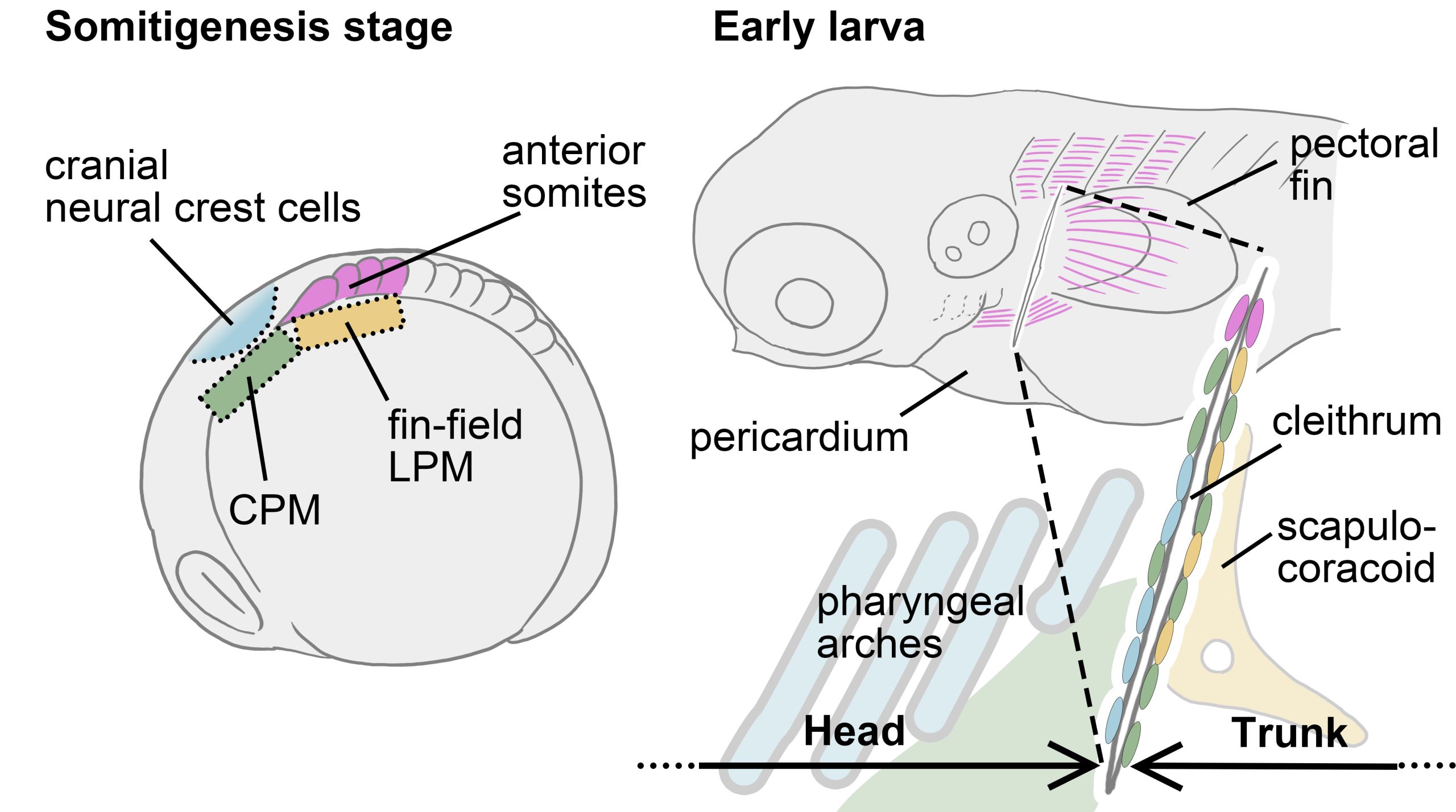
We have now successfully mapped the four distinct cell populations onto the cleithrum, from somitogenesis embryo to early larva. Let’s take another look at the development and evolution of the pectoral/shoulder girdle with this map in hand. First, the cleithrum indeed shared a developmental origin with the fin-field LPM, which differentiates into the scapulocoracoid. Perhaps the phenotype reported in the gli3 mutant mentioned above is related to the signal responsiveness of the embryonic resources shared by the cleithrum and scapulocoracoid, such as the LPM. If changes in Hedgehog-Gli signaling were also involved in the tetrapod evolution, the cleithrum may have degenerated and eventually disappeared due to losing the “resource competition” with the scapulocoracoid.
Indeed, a hypothesis has been proposed that not just a part of the LPM-derived cleithrum, but the entire cleithrum, was incorporated into the mammalian scapula through evolution [Matsuoka et al., 2005]. However, our results did not perfectly support this hypothesis. This is because recent results of genetic cell lineage analysis using mice could not confirm the contribution of neural crest cells and CPM (collectively referred as head mesenchyme) to the scapula [Adachi et al., 2020; Heude et al., 2018], while these head mesenchyme contributes to the cleithrum in zebrafish. Given this mismatch of embryonic origins of mammalian shoulder and fish pectoral girdle, our view is that the head mesenchyme which would have formed the cleithrum in ancestral tetrapods, no longer participates in the formation of the shoulder girdle skeleton in derived amniotes. This rearrangement of cell populations at the head/trunk interface may be due to the evolution of a specialized LPM region, which should be called the “neck LPM”, required for the formation of the long neck characteristic of the modern amniotes [Hirasawa et al., 2016; Lours & Dietrich, 2005]. This novel LPM population may have spatially separated the cranial mesenchyme that would have formed the cleithrum in ancestral animals from the trunk mesenchyme, leading to a cleithrum loss in multiple lineages.
Remaining questions
We propose that if we assume the complex lineages that make up the cleithrum is mechanistically indispensable for its formation, we can reasonably explain the evolutional acquisition of the functional neck and the concurrent disappearance of the cleithrum in amniotes. However, it is not yet clear what role each cell lineages plays in the formation of the cleithrum. If one or two of the four cell populations that make up the cleithrum are missing, can the other cell populations compensate for the absence of the missing cell populations and still form the cleithrum? If so, are the developmental origins of animals with the cleithra diverse? Furthermore, we emphasize that the evolution of the cleithrum and the neck cannot be discussed separately. So, then, what exactly is the neck LPM in amniotes? When and how did it evolve through changes in the ancestral developmental system? Some of these questions are now being actively pursued in the Nakamura lab, and of course, are open questions for all researchers interested in the evolution of the shoulder and neck as well.
References
Adachi, N., Bilio, M., Baldini, A., & Kelly, R. G. (2020). Cardiopharyngeal mesoderm origins of musculoskeletal and connective tissues in the mammalian pharynx. Development, 147(3), dev185256. https://doi.org/10.1242/dev.185256
Cole, N. J., Hall, T. E., Don, E. K., Berger, S., Boisvert, C. A., Neyt, C., Ericsson, R., Joss, J., Gurevich, D. B., & Currie, P. D. (2011). Development and evolution of the muscles of the pelvic fin. PLoS Biol, 9(10), e1001168. https://doi.org/10.1371/journal.pbio.1001168
Gegenbaur, C. (1895). Clavicula und Cleithrum. Morphologisches Jahrbuch, 23, 1-20.
Gess, R., & Ahlberg, P. E. (2018). A tetrapod fauna from within the Devonian Antarctic Circle. Science, 360(6393), 1120-1124. https://doi.org/10.1126/science.aaq1645
Heude, E., Tesarova, M., Sefton, E. M., Jullian, E., Adachi, N., Grimaldi, A., Zikmund, T., Kaiser, J., Kardon, G., Kelly, R. G., & Tajbakhsh, S. (2018). Unique morphogenetic signatures define mammalian neck muscles and associated connective tissues. Elife, 7, e40179. https://doi.org/10.7554/eLife.40179
Hirasawa, T., Fujimoto, S., & Kuratani, S. (2016). Expansion of the neck reconstituted the shoulder-diaphragm in amniote evolution. Development, Growth & Differentiation, 58(1), 143-153. https://doi.org/10.1111/dgd.12243
Jarvik, E. (1980). Basic structure and evolution of vertebrates (Vol. 1). Academic Press. https://books.google.co.jp/books?id=UMwKAQAAIAAJ
Kuroda, S., Lalonde, R. L., Mansour, T. A., Mosimann, C., & Nakamura, T. (2024). Multiple embryonic sources converge to form the pectoral girdle skeleton in zebrafish. Nature Communications, 15(1), 6313. https://doi.org/10.1038/s41467-024-50734-x
Lours, C., & Dietrich, S. (2005). The dissociation of the Fgf-feedback loop controls the limbless state of the neck. Development, 132(24), 5553-5564. https://doi.org/10.1242/dev.02164
Matsuoka, T., Ahlberg, P. E., Kessaris, N., Iannarelli, P., Dennehy, U., Richardson, W. D., McMahon, A. P., & Koentges, G. (2005). Neural crest origins of the neck and shoulder. Nature, 436(7049), 347-355. https://doi.org/10.1038/nature03837
Nakamura, T., Gehrke, A. R., Lemberg, J., Szymaszek, J., & Shubin, N. H. (2016). Digits and fin rays share common developmental histories. Nature, 537(7619), 225-228. https://doi.org/10.1038/nature19322
Romer, A. S., & Parsons, T. S. (1986). The vertebrate body (6th ed.). Saunders College.
Shimada, A., Kawanishi, T., Kaneko, T., Yoshihara, H., Yano, T., Inohaya, K., Kinoshita, M., Kamei, Y., Tamura, K., & Takeda, H. (2013). Trunk exoskeleton in teleosts is mesodermal in origin. Nature Communications, 4(1), 1639. https://doi.org/10.1038/ncomms2643
Vogt, W. (1929). Gestaltungsanalyse am Amphibienkeim mit örtlicher Vitalfärbung.II. Teil Gestaltungsanalyse am Amphibienkeim mit Örtlicher Vitalfärbung. Wilhelm Roux’ Archiv für Entwicklungsmechanik der Organismen, 120, 384-706. https://doi.org/10.1007/BF02109667
Wei, J., Wood, T. W. P., Flaherty, K., Enny, A., Andrescavage, A., Brazer, D., Navon, D., Stewart, T. A., Cohen, H., Shanabag, A., Kuroda, S., Braasch, I., & Nakamura, T. (2023). Distinct ossification trade-offs illuminate the shoulder girdle reconfiguration at the water-to-land transition. bioRxiv, 2023.2007.2017.547998. https://doi.org/10.1101/2023.07.17.547998