Sex combs in motion
Posted by JNMalagon, on 14 November 2018
Using computer simulations and mathematical modeling to study the evolution of morphogenesis
Juan N. Malagon and Ernest Ho tell the story behind their recent paper in PLOS Computational Biology.
In the Larsen lab, we are interested in testing a 50-year old question: How do sex combs rotate in fruit flies? Despite extensive studies of the process using 4D confocal microscopy, there remain many questions about the spatial and temporal dynamics of sex comb rotation we cannot address with currently available tools. We therefore turned to computer simulations and mathematical modelling to explore these issues.
It was love at first sight. JNM: I came to the University of Toronto with a strong interest in understanding how physical forces influence the evolution of morphogenesis. My PhD supervisor, Ellen Larsen, showed me a perfect model to explore this biological question: sex comb rotation. Although I was a bit skeptical because I did not know what a sex comb was and did not have any experience working with fruit flies, she convinced me in less than one minute. To do so, she showed a time-lapse movie of the rotating comb made by the other student in the lab, Joel Atallah (Mov. 1A). I was so intrigued by the beauty of this morphogenetic process, it was love at first sight.
Movie 1. Sex comb rotation. A) Time-lapse movie B) simulation. Early (red polygons) and late cell expansion (blue polygons). Rotating comb (green polygons), epithelial cells above the comb (yellow polygons).
Our hands were tied. This movie shows the development of the fore leg epithelium and embedded within it, a rotating row of bristle cells, the sex comb (Mov. 1). Thanks to the genetic tools available for fruit flies, we imaged combs of different lengths and angles of rotation. After a few years of hard work, we proposed that the driving force for the rotation came from a push from the epithelial cell growth underneath the sex comb1. However, to study cell growth in local areas and at different times was impossible with available biological techniques. Although our hands were tied using experimental biological tools, computer simulations set us free to explore the development and evolution of this morphogenetic process. To give you more insight into the comb rotation problem, here is some background information.
What are the sex combs of fruit flies?
Sex combs are male-specific group(s) of bristles located on the front legs of many Drosophila species (Fig. 1).
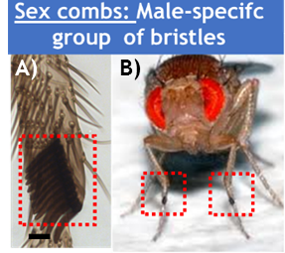
This row of bristles is used during courtship behavior, increasing the quantity of successful matings. As with any sexual trait, sex combs display an incredible morphological diversity among Drosophila species (Fig. 2).
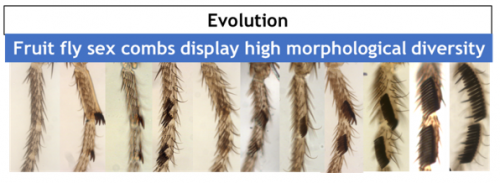
Why study sex comb rotation? Despite the rapid progress achieved in understanding the genetic basis of evolution, the cellular basis remains much less studied. Sex combs are a great tool to investigate the cellular basis of evolution. Biologists including in our laboratory have used sex combs to study the developmental mechanisms underlying biodiversity1 and the cellular basis of allometric evolution2. Our present work shows an example in which mechanical forces play a fundamental role in understanding the evolution of morphogenesis.
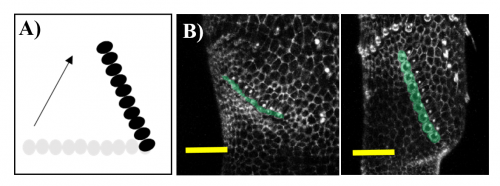
A bit of history. The study of sex comb rotation commenced in 1962 when Chiyoko Tokunaga, using genetic mosaics produced in sex combs during different times in development, hypothesized that this group of bristle cells changes in position during development3 (Fig 3A).
Our laboratory has concentrated on developing and standardizing an in vivo imaging technique to study comb rotation. To investigate the cellular mechanisms involved, we combine this imaging technique with standard genetic tools available for studying fruit fly morphogenesis such as mutants, transgenic flies with fluorescent proteins and artificial selection. Our work led to three candidate models concerning the source of the force underlying rotation (Fig. 4).
The one which hypothesizes the source of pushing force coming from the region distal to the sex comb (the “push” model) appears to best explain existing experimental data.
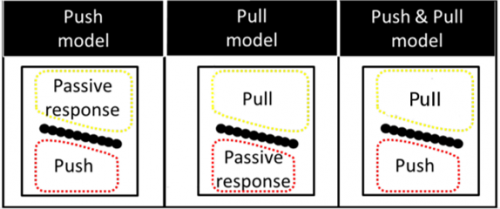
United we stand. Even though we have evidence to support the “push” model of sex comb rotation, we are still lacking the critical insight on how the apparent random nature of distal epithelial cell expansion can properly rotate the comb. We believe that mathematics can help solve the mystery.
EH: I am a physicist and neuroscientist by training, and currently a data scientist by profession. Juan and myself were dormmates for several years at the University of Toronto. Over many dinner-table conversations, we discovered we shared a common interest in using mathematics to understand biology. After getting my PhD in computational neuroscience, I went on to do a post-doc in epilepsy research and had also been involved with industrial data science projects. While juggling my outside data science gigs, I collaborated with Juan and developed a mathematical model describing sex comb rotation. Computer simulations derived from the mathematical model (made easy with the open-source package compucell3d5 and a modern high-performance computing facility6) were used to mimic the cell properties of the developing comb (Mov. 1B and Fig. 5).
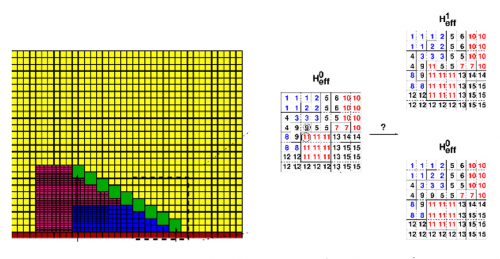
A picture of sex comb rotation finally emerges as a result of this computational-experimental approach. The picture clearly depicts how the underlying epithelial cell dynamics facilitate sex comb rotation—by utilizing the asymmetry in the timing and location of cell growth. First, the epithelial cells start out smaller closer to the leading tip of the comb as compared to the comb base, thus creating an inhomogeneity in initial cell size distribution (Fig. 6).
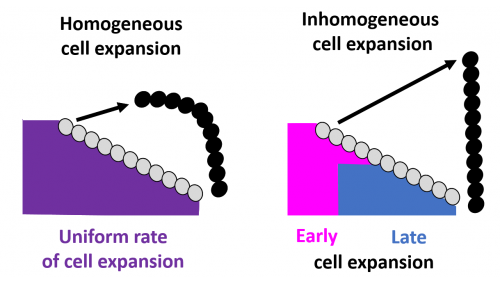
As the cells expand, this inhomogeneity translates into a differential push which maintains the shape of the comb during the entire rotation. In addition, the mathematical model identified a temporal component in which delayed expansion of distal cells closer to the comb base protects the combs from breaking during rotation, a phenomenon also subsequently verified by experimental imaging data.
We will discuss below how our discoveries have shed light on the essential relationship between comb evolution and its underlying cellular processes.
Evolutionary implications
How to modify comb orientation. Sex combs in the family Drosophilidae can be divided into three main groups based on their orientation relative to the joint: transverse (0˚-30˚), diagonal (30˚-60˚), and vertical (60˚-90˚) (Fig. 7).
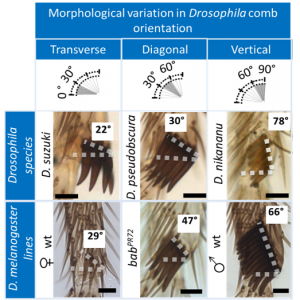
Phylogenetic analyses reveal that changes in comb orientation not only occur frequently, but also occur between closely related Drosophila species. To study how comb orientation evolves, we used genetic perturbations in D. melanogaster that phenocopy the morphological variations in nature (Mov. 2 and Fig. 7).
Movie 2. Time-lapse movies of D. melanogaster lines with three comb orientations.
In vivo experiments show that a reduction in apical cell expansion leads to a reduction in the angle of rotation. With computer simulations, we now understand that nature exploits the asymmetry of distal cell expansion to achieve this goal. In other words, the smaller the degree of rotation, the smaller the degree the inhomogeneity in cell expansion is required for the rotation. These findings suggest that changes in comb orientation can occur via fine-tuning the pattern of cell growth to achieve the pattern of inhomogeneity we have described. This narrows our focus to determining those genes and tissue forces involved, which will go far in understanding the enigma of sex comb rotation and perhaps other morphogenetic events where local differential growth occurs.
Relationship between comb length and shape. Despite the spectacular morphological diversity observed in Drosophila combs, their shape tends to be straight (Fig. 8).
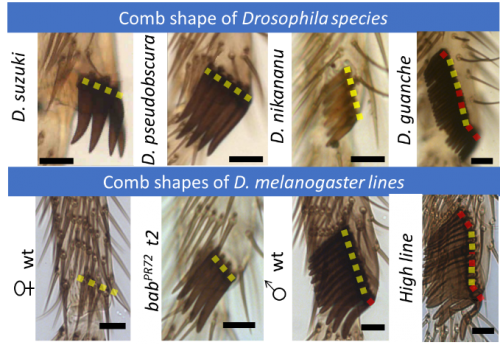
Since the combs are used as a grasping tool, a straight comb shape seems to be fundamental for proper functioning. Our computational model uncovers the critical mechanisms that maintain the comb shape during the entire rotation.
Experimentally, short combs tend to be straight while long combs are more likely to display minor bends (Fig. 9).
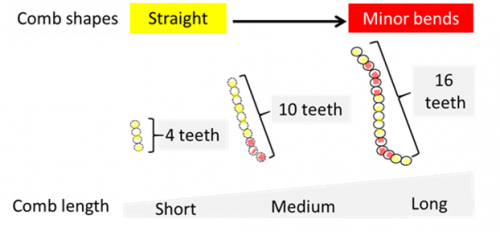
In fact, computer simulations show that increasing comb length is associated with multiple mechanical limitations to achieve a proper rotation. Long combs, for instance, are more likely to break during rotation (Mov. 3).
Movie 3. Long combs are more likely to break during morphogenesis. Examples of adult legs displaying sex comb breaking in Drosophila species (A) and Drosophila melanogaster lines (B). Schematic (C) and computer simulation (D) showing sex comb breaking during rotation.
These findings are consistent with the Drosophila comb diversity observed in nature. Most of the long combs (>15 bristles) display a different mechanism to achieve a vertical position. The only exception is Drosophila guanche, but as predicted by our model, this Drosophila comb generally displays bent and broken phenotypes.
Future directions. This work shows that the computational-experimental approach is an effective method to study morphogenesis and its evolution. For example, we can use simulations to further explore the thresholds beyond which long combs are unable to rotate due a spatial limitation1. The computer simulations on sex comb rotation generated many hypotheses that will be tested by combinations of new techniques.
References
- Malagon JN, Ahuja A, Sivapatham G, Hung J, Lee J, Muñoz-Gómez S, et al. Evolution of Drosophila sex comb length illustrates the inextricable interplay between selection and variation. PNAS.;111(39):E4103–E4109. (2014) pmid:25197080
- Malagon, J. N. & Khan, W. Evolution of allometric changes in fruit fly legs: A developmentally entrenched story. Acta Biol. Col. 21, (3):509-519 (2016).
- Tokunaga, C. Cell Lineage and Differentiation on the Male Drosophila melanogaster Foreleg of Drosophila melanogaster. Dev. Bio. (4), 489–516 (1962).
- https://www.cs.mcgill.ca/~rwest/wikispeedia/wpcd/images/178/17885.jpg.htm
- Swat M, Thomas G, Belmonte J, Shirinifard A, Hmeljak D, Glazier J. Multi-Scale Modeling of Tissues Using CompuCell3D. Methods in Cell Biology. (110), 325–366 (2012) pmid:22482955
- https://www.westgrid.ca
Acknowledgements. We are deeply grateful to Joel Atallah, Lewis Held and Artyom Kopp for their detailed and constructive comments during this challenging project.
Juan N. Malagon is currently a Research Associate with Dr. Ellen Larsen
and hopes to get a faculty position, which allows him to combine his
two passions: teaching and developing research projects with students. His professional website is: https://juannicolasmalagon.com.
Ernest Ho is currently working with neuro-informatics projects at the Ontario Brain Institute. He hopes to work with data science or mathematical modelling projects either in academia or industry. His LinkedIn profile is: https://www.linkedin.com/in/ehocanada. His ResearchGate profile is: https://www.researchgate.net/profile/Ernest_Ho.