Exploring the differences between mice and men during cerebral cortex development
Posted by Gavin Clowry, on 5 December 2017
Alzu’bi A, Lindsay SJ, Harkin LF, McIntyre J, Lisgo SN, Clowry GJ (2017) The transcription factors COUP-TFI and COUP-TFII have distinct roles in arealisation and GABAergic interneuron specification in the early human fetal telencephalon. Cerebral Cortex 27: 4677-4690.
One author’s perspective on the origins of this study (Gavin Clowry)
For me the origins of this research paper stretch back nearly twenty years. I was part of a research group, led by the paediatric neurologist Professor Janet Eyre, studying the neurobiology of cerebral palsy including neurophysiological studies on infants and children. A little boy with hemiplegia generated some remarkable results. He was unusual in that he had suffered a brain lesion relatively early in development compared to most CP sufferers, around mid-gestation, resulting in destruction of the sensorimotor cortex unilaterally. Transcranial magnetic stimulation, coupled with electromyographic recording, showed that muscles contralateral to the lesion were strongly responsive to stimulation of the ipsilateral motor cortex, not unusual in hemiplegics. What was unusual, though, was the strong response of the same muscles to stimulation of the intact contralateral occipital cortex. Ten more years of experiments and imaging were required to confirm these observations [1] but it was shown that in response to the lesion, visual cortex had acquired or maintained projections to motor centres in the spinal cord (Figure 1).
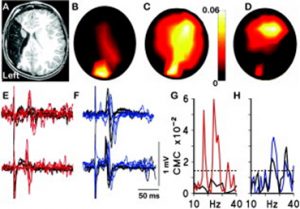
This set us speculating on the nature and plasticity of the protomap in human. Around this time strong evidence was being presented in favour of the protomap hypothesis originally proposed by Pasko Rakic [2]. The hypothesis stated that the organisation of the cerebral cortex into functional areas is determined by the co-ordinated and compartmented expression of genes in time and space at the earliest stages of its development, prior to its connection with the sensory input that could drive its maturation. Experiments in mice (Figure 2) [3, 4] were showing that altered gradients of transcription factor expression across the early cortex in mutant and transgenic mice led to an altered functional map for primary cortical areas. But does all this hold true for human development? For our next programme of research we teamed up with Prof Susan Lindsay and the Human Developmental Biology Resource to explore gene expression in the early stages of human cortex development especially to look for evidence for a human protomap.
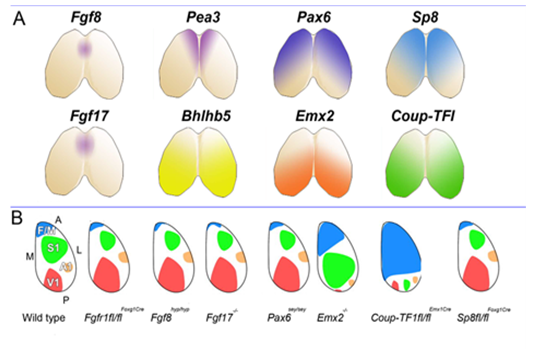
Our first success was to show that the transcription factors PAX6 and EMX2 form counter-gradients across the human cortex, just as they do in mouse, but only at the very earliest stages of cortical plate formation (Figure 3) [6]. Unlike in mouse, where expression is confined to progenitor cells, EMX2 was found to be expressed in a gradient in the post-mitotic neurons of the cortical plate as well. We also found arealised expression for other genes, by histological techniques, from quantitative measurements of mRNA expression, and in fetal cells cultured from different cortical regions [7, 8]. For instance, the layer V specific transcription factor CTIP2, along with transmembrane signalling molecules and corticospinal tract markers ROBO1 and SRGAP1, are more highly expressed in the frontal cortex between 9-12 PCW which might represent the predominance of corticofugal projection neurons in frontal sensorimotor areas.
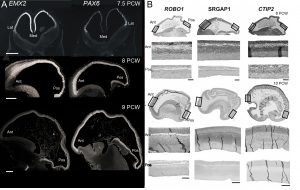
However the crucial question is whether such a developmental scheme observed in rodents is sufficient to produce all the extra areas of specialised association cortex, along with the vastly increased interconnectivity between these areas, seen in primates [9]? We believe our latest study may shed some light on this question [10]. We studied expression patterns of the arealising transcription factors COUP-TFI, COUP-TFII and SP8. What was noticeable in humans was that SP8 and COUP-TFI overlap extensively in the ventricular zone of visual, auditory and somatosensory cortex. This differs from the mouse in which COUP-TFI and SP8 show little overlap. Combinatorial expression of COUP-TFI and SP8 could maintain a common genetic identity for future primary sensory areas (visual, auditory and somatosensory) and a partially shared identity with SP8-expressing frontal motor cortex with which these sensory areas will interconnect, along with allied association cortex, via dorsal sensorimotor pathways. In mouse COUP-TFII is confined to a very small portion of the posterior cortex but in human is expressed extensively throughout the ventral temporal and ventral posterior cortex where it overlaps with COUP-TFI expression. Perhaps the expansion of cortical COUP-TFII expressing territory in human fetal brain mirrors the increased size and complexity of the association areas of the ventro-temporal cortex. An extension of this observation is that dorsal and ventral hippocampus are also differentiated by combinatorial expression of SP8/COUP-TFI and COUP-TFII/COUP-TFI respectively. Each domain has distinct functions and distinct efferent and afferent connections. It appears that the protomap for human hippocampal specialisation is laid down early in development.
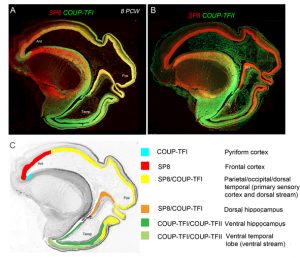
(A) Expression of opposing gradients of SP8 and COUP-TFI in a sagittal section of human fetal telencephalon at 8 Post-conceptional weeks. (B) compartmentalised expression of SP8 and COUP-TFII in the developing cerebral cortex. (C) summarises the findings in A and B demonstrating how the progenitor zones of the cortex are subdivided into compartments by transcription factor expression that give rise to different functional areas of cortex in maturity.
It has been a 15 year journey to reach the beautiful images in Figure 4 with many interesting diversions along the way; for instance, when studying PAX6 expression we discovered a new class of progenitor cell in the subventricular zone of the cortex [11] which was subsequently shown to be a new class of radial glia [12]. We now know that humans as well as rodents have a protomap to guide cortical development, but this protomap is plastic and can be perturbed by genetic alteration, pharmacological interventions and lesions. Although more investigation is needed, it seems highly likely that the protomap has evolved in complexity as the cerebral cortex has evolved in complexity, rather than entirely new mechanisms being required to shape the cortex’s functional organisation, although, as ever, more research is needed to test this assertion. Ensuring that neurons in the cortex have the right regional and functional identity is crucial to establishing their long and short range connectivity, deficits in which are proposed to underlie neurodevelopmental orders such as autism and schizophrenia, so there is good reason to extend this journey a while longer yet.
References
- Basu A, Graziadio S, Smith M, Clowry GJ, Cioni G, Eyre JA (2010) Developmental plasticity connects visual cortex to motoneurons after stroke. Annals of Neurology 67:132-6.
- Rakic P (1988) Specification of cerebral cortical areas. Science 241:170.
- Bishop KM, Goudreau G, O’Leary DDM (2000) Regulation of area identity in the mammalian neocortex by Emx2 and Pax6. Science 288:344-9.
- Muzio L, Di Benedetto B, Stoykova A, Boncinelli E, Gruss P, Mallamaci A (2002) Emx2 and Pax6 control regionalization of the pre-neuronogenic cortical primordium. Cerebral Cortex 12:129-39.
- Clowry GJ, Alzu’bi A, Harkin LF, Sarma S, Kerwin J, Lindsay S (2017) Charting the protomap of the human telencephalon. Seminars in Cell and Developmental Biology, Epub ahead of print, doi.org/10.1016/j.semcdb.2017.08.33.
- Bayatti N, Sarma S, Shaw C, Eyre JA, Vouyiouklis DA, et al (2008) Progressive loss of PAX6, TBR2, NEUROD and TBR1 mRNA gradients correlates with translocation of EMX2 to the cortical plate during human cortical development. European Journal of Neuroscience 28: 1449-1456.
- Ip BK, Wappler I, Peters H, Lindsay S, Clowry GJ, Bayatti N (2010) Investigating gradients of gene expression involved in early human cortical development. Journal of Anatomy 217: 300-311.
- Ip BK, Bayatti N, Howard NJ, Lindsay S, Clowry GJ (2011) The corticofugal neuron-associated genes ROBO1, SRGAP1, and CTIP2 exhibit an anterior to posterior gradient of expression in early fetal human neocortex development. Cerebral Cortex 21: 1395-1407.
- Buckner RL, Krienen FM (2013) The evolution of distributed association networks in the human brain. Trends in Cognitive Sciences 17: 648-65.
- Alzu’bi A, Lindsay SJ, Harkin LF, McIntyre J, Lisgo SN, Clowry GJ (2017) The transcription factors COUP-TFI and COUP-TFII have distinct roles in arealisation and GABAergic interneuron specification in the early human fetal telencephalon. Cerebral Cortex 27: 4677-4690.
- Bayatti N, Moss JA, Sun L, Ambrose P, Ward JFH, Lindsay S, Clowry GJ (2008) A molecular neuroanatomical study of the developing human neocortex from 8 to 17 postconceptional weeks revealing the early differentiation of the subplate and subventricular zone. Cerebral Cortex 18: 1536-1548.
- Lui, J.H. Hansen, D.V., Kriegstein, A.R. 2011. Development and evolution of the human neocortex. Cell 146: 18–36.