Kink in the road: the notochord’s role in spine formation and scoliosis
Posted by Jennifer Bagwell, on 14 April 2020
By Jennifer Bagwell and Michel Bagnat
Our lab investigates the role of hydrostatic pressure as a morphogenetic force using zebrafish as a model system. This work was originally focused on tube formation and had revealed a crucial role for fluid secretion in single lumen formation (Navis and Bagnat, 2015). When I joined, the lab was conducting a forward genetic screen looking for phenotypes involving fluid regulation. We found many mutants with defects in the anterior-posterior (AP) axis and this brought our attention to the notochord. The notochord is the central structural element in the AP axis of all chordates and in vertebrates it serves as scaffold for the spine. In the developing vertebrate embryo, the notochord acts as a hydrostatic skeleton (Adams et al., 1990) and is important in the patterning of several tissues including the somites, blood vessels and pancreas. While the exact morphology of the notochord can differ between species, one key feature is highly conserved: the presence of large, fluid-filled vacuolated cells, also known as chordocytes (Grotmol et al., 2003). These cells are found in all vertebrate embryos and they persist beyond early development, being found in the nucleus pulposus of the intervertebral disks in mice, pigs, and humans (Lawson and Harfe, 2015). Their most salient feature is that the vast majority of their cell volume is occupied by a giant (~40µm) fluid-filled vacuole (Fig 1).
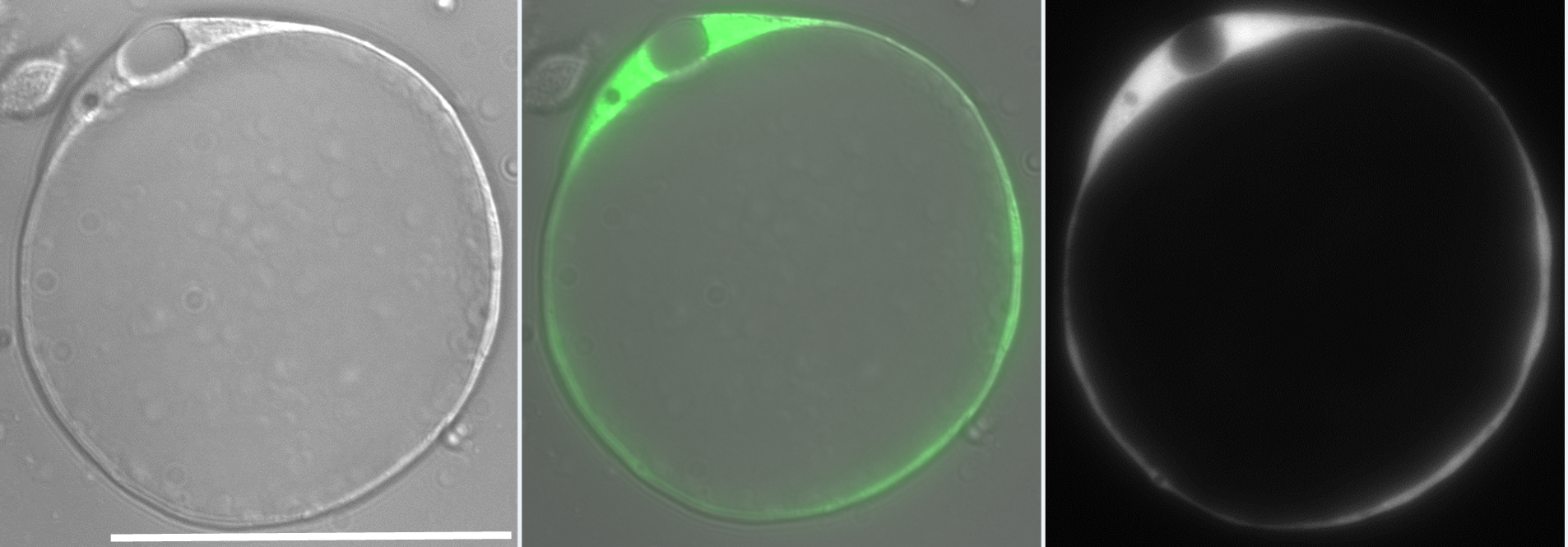
The zebrafish notochord is comprised of three parts: a core of large vacuolated cells; a layer of epithelial-like sheath cells that surrounds the core; and a thick extracellular matrix encasing the sheath cells. (Ellis et al., 2013b) (Fig 2.). When we started working on the notochord, we first wanted to define the identity of its unusual vacuoles. Combining a genetic and cell biological approach, we showed that notochord vacuoles are indeed lysosome related organelles which form via post-golgi biosynthetic trafficking and that they are crucial for embryonic axis elongation (Ellis et al., 2013a). Surprisingly, we also found that when vacuoles are disrupted during early development spine formation is also affected, causing a phenotype reminiscent of scoliosis in humans (Ellis et al., 2013a). Scoliosis is the most common developmental disorder of the spine and results in kinks and curvatures of the spines axis. Seeing spine malformations was a very exciting result for us and led us down a new road to understand how the notochord functions during spine morphogenesis.
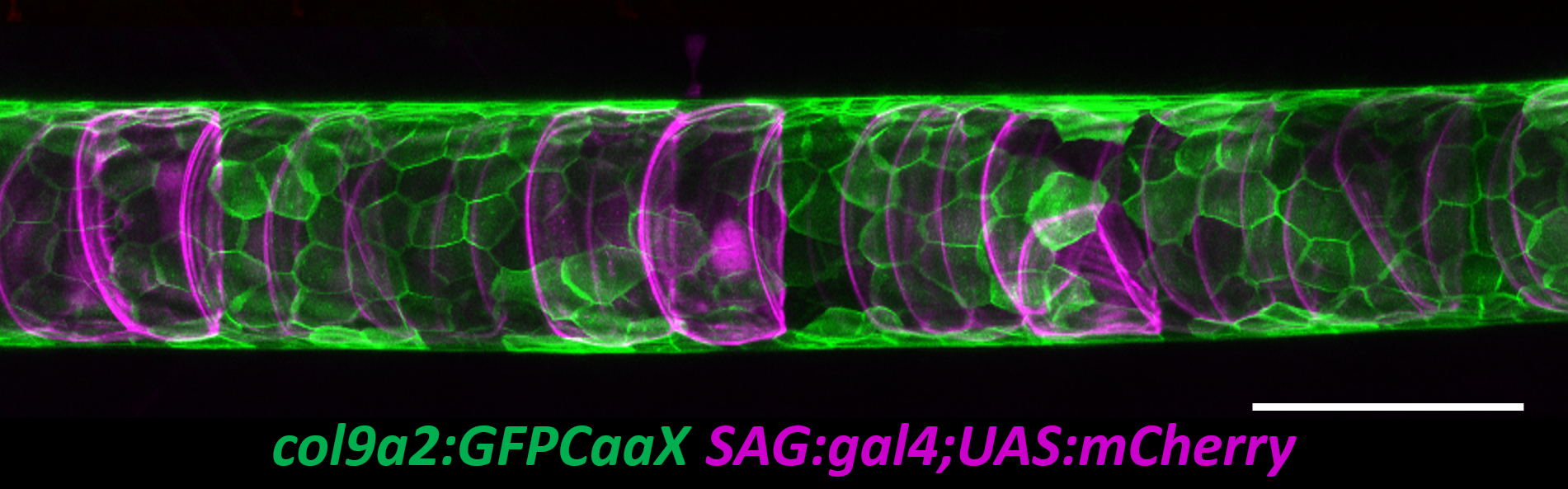
To tackle this question, I started with a live imaging approach. We spent a lot of time generating transgenic lines to visualize the different cell types of the notochord and spine and learning how to image fish during late larval stages. While imaging the early embryo with confocal microscopy is relatively easy, imaging the notochord during spine development proved to be much more difficult. The tissue is thicker, external pigment develops, and it is much harder to keep the fish alive while on the scope. However, after a lot of trial and error we developed an imaging protocol that allowed us to image spine formation until about 6 weeks post fertilization. We also developed quantitative methods to determine the exact shape and position of every vacuolated cell in the notochord (Norman et al., 2018).
It soon became clear that vacuolated cells and their vacuoles persist through spine morphogenesis. Interestingly, we found that the morphology and arrangement of vacuolated cells changes during development. Vacuolated cells that remain under the growing vertebra change in shape from a rounded morphology to an elongated, pancake-like morphology; whereas vacuolated cells that end up in IVD (intervertebral disk) areas become tightly packed and their vacuoles fragment (Bagwell et al., 2020). It appeared as though the vacuolated cells are squished under the concentrically growing vertebrae, but are more rounded and clumped together in the IVD domains. This led us to hypothesize that the vacuoles are important in providing a scaffold that is able to initially resist the pressure of the growing vertebrae, symmetrically and consistently, allowing for the development of a straight spine (Fig. 3 A,C,E).
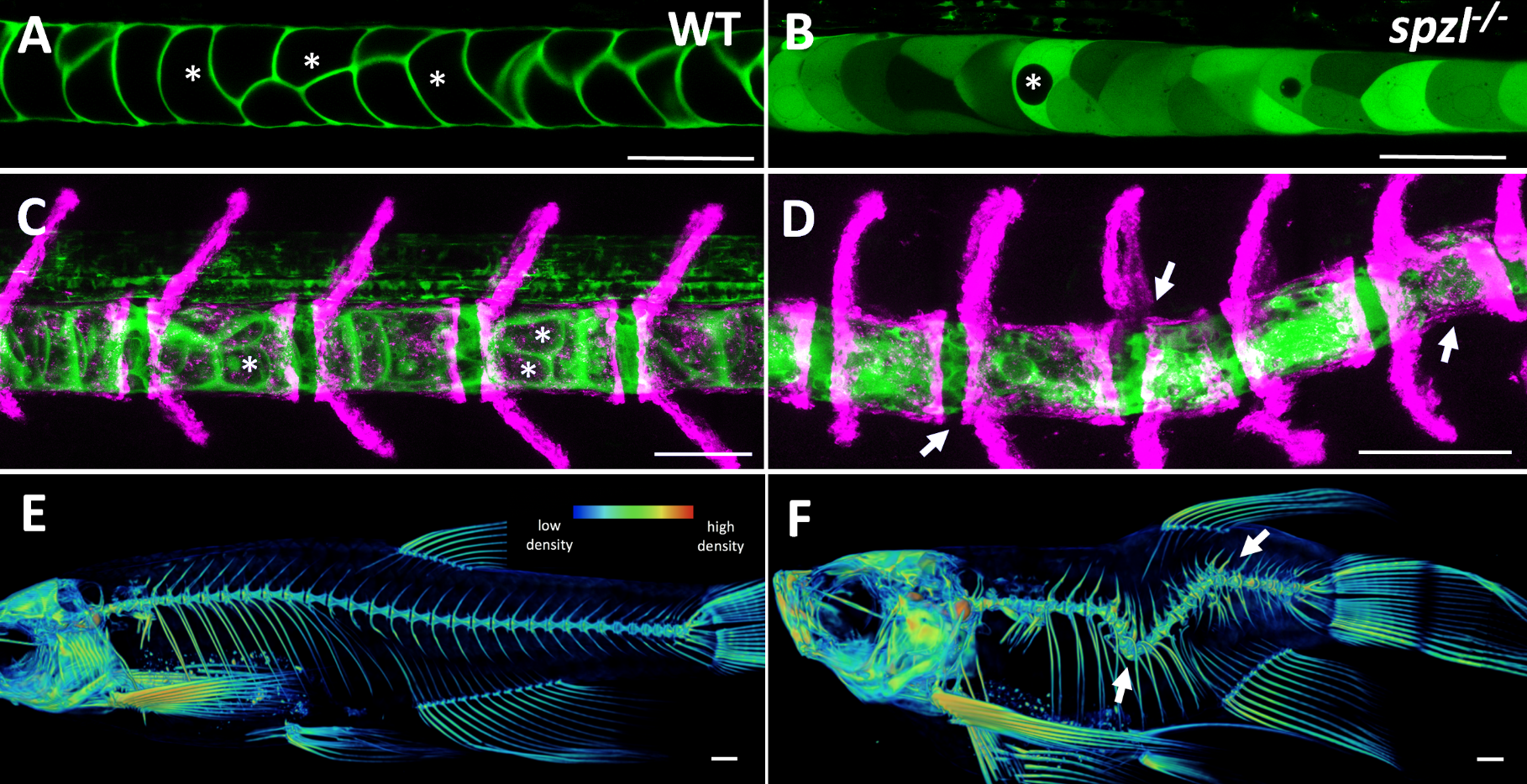
At this point in the project, we were incredibly fortunate to collaborate with Didier Stanier, whose lab had carried out a forward genetic screen to identify mutants with defects in endodermal organs. They had found, by chance, a viable mutant with a shortened embryonic axis and what appeared to be a severe scoliosis phenotype. Because Didier knew we were working on understanding spine morphogenesis and the origins of scoliosis, he sent the mutant to our lab. This little mutant was so twisted and short, we affectionately named it spaetzle (spzl) after the German pasta (incidentally, Didier’s lab moved to Germany shortly after that). Right away we saw that the vacuoles in spzl were smaller and fragmented, and live imaging revealed that the vacuoles continued to deteriorate throughout development, leaving intact cells with really small or no vacuoles at all. We were surprised to find that the notochord and the AP axis were initially straight in spzl mutants. However, as vertebra formed the notochord was unevenly deformed, and severe kinking ensued as the bone grew (Bagwell et al., 2020). It was then clear that this mutant was a great genetic model to investigate the role of notochord vacuoles in spine formation.
The first step for experimentally testing our ideas was to locally disrupt vacuoles to see if spine curvatures or kinks would occur at those specific locations. Simple right? There are many tools ablate cells including nitroreductase lines, photo ablation and physical injury, just to name a few. We had nitroreductase transgene perfect for this experiment and we could express it mosaically, kill a few cells and then see what happens with the spine. So I tried it successfully killing the cells, raised them to juvenile stages, and imaged their spines, but found no scoliosis. I was puzzled: how could we have such a strong phenotype in our spzl mutant, but be unable to reproduce the phenotype experimentally? Around this time Jamie Garcia, a graduate student, joined the lab and “Team Notochord”. She was looking at the role of cup shaped invaginations called caveolae, which the Parton lab had shown to be incredibly abundant in the notochord (Nixon et al., 2007). In parallel studies, Jamie and I on one hand (Garcia et al., 2017), and the Parton lab on their own (Lim et al., 2017), showed that upon locomotion, vacuolated cells lacking caveolae collapse, causing lesions in the notochord. We then found that as the vacuoles burst they release their contents, particularly ATP and UTP, which induce notochord sheath cells to locally invade the notochord core, transdifferentiate, and vacuolate, thus restoring the integrity of the notochord and allowing for a straight spine (Garcia et al., 2017). This finally explained why mutants lacking caveolae did not have a spine phenotype and why all the ablation assays I tried and tried did not work.
This ability of the notochord to regenerate vacuolated cells upon physical or mechanical injury reinvigorated our hypothesis that vacuolated cells are critical for spine development. I thought that if the notochord has the ability to fix itself, it must be important for later development. While vacuoles are lost in the spzl mutant, vacuolated cells remain intact and do not induce any sheath cell invasion or vacuolated cell regeneration. Through light sheet microscopy, I showed that spzl mutants are unable to undergo vesicle fusion to the vacuole membrane and are therefore unable to make and maintain a fully inflated vacuole, leaving a notochord that is lacking an even distribution of fully inflated vacuoles. Using nuclear deformation assay that James Norman and I developed, we also found that loss of vacuole integrity reduces the internal pressure of the notochord. Then, as vertebrae begin growing and squeezing the notochord, the structure deforms irregularly and is unable to absorb compression evenly. As a result, asymmetric deformations develop along the notochord, ultimately leading to severe kinking of the spine axis (Bagwell et al., 2020) (Fig 3 B,D,F). We were also able to recapitulate the mechanism with an experimental manipulation by expressing a dominant-negative form of the GTPase Rab32a. We showed that mosaic expression of DN-GFPRab32a causes vacuole fragmentation and local spine deformation at juvenile stages, further confirming our hypothesis. With the help of Sarah McMenamin’s lab we were also able to show using high-resolution micro CT imaging that loss of notochord vacuoles also leads to increased vertebral bone mineralization, suggesting notochord stiffness and vertebral bone formation are cross-regulated (Bagwell et al., 2020).
Our notochord journey started about 10 years ago. Our path began by asking two simple questions: what are notochord vacuoles and what are they good for? Like many questions in science this has led us down a road with lots of forks, turns and roadblocks. The forks in our path have led us to many new and exciting stories about the notochord such as its role in spine segmentation (Wopat et al., 2018), and there are many more to come. We have learned so much about this simple yet dynamic structure, but what is clear is that there is still so much more to learn. In the words of Yogi Berra
“Keep trying, stay humble, trust your instincts, most importantly, act. When you come to a fork in the road, take it.”
References
Adams, D.S., Keller, R., Koehl, M.A., 1990. The mechanics of notochord elongation, straightening and stiffening in the embryo of Xenopus laevis. Development 110, 115-130.
Bagwell, J., Norman, J., Ellis, K., Peskin, B., Hwang, J., Ge, X., Nguyen, S.V., McMenamin, S.K., Stainier, D.Y., Bagnat, M., 2020. Notochord vacuoles absorb compressive bone growth during zebrafish spine formation. Elife 9.
Ellis, K., Bagwell, J., Bagnat, M., 2013a. Notochord vacuoles are lysosome-related organelles that function in axis and spine morphogenesis. J Cell Biol 200, 667-679.
Ellis, K., Hoffman, B.D., Bagnat, M., 2013b. The vacuole within: how cellular organization dictates notochord function. Bioarchitecture 3, 64-68.
Garcia, J., Bagwell, J., Njaine, B., Norman, J., Levic, D.S., Wopat, S., Miller, S.E., Liu, X., Locasale, J.W., Stainier, D.Y.R., Bagnat, M., 2017. Sheath Cell Invasion and Trans-differentiation Repair Mechanical Damage Caused by Loss of Caveolae in the Zebrafish Notochord. Curr Biol 27, 1982-1989 e1983.
Grotmol, S., Kryvi, H., Nordvik, K., Totland, G.K., 2003. Notochord segmentation may lay down the pathway for the development of the vertebral bodies in the Atlantic salmon. Anat Embryol (Berl) 207, 263-272.
Lawson, L., Harfe, B.D., 2015. Notochord to Nucleus Pulposus Transition. Curr Osteoporos Rep 13, 336-341.
Lim, Y.W., Lo, H.P., Ferguson, C., Martel, N., Giacomotto, J., Gomez, G.A., Yap, A.S., Hall, T.E., Parton, R.G., 2017. Caveolae Protect Notochord Cells against Catastrophic Mechanical Failure during Development. Curr Biol 27, 1968-1981 e1967.
Navis, A., Bagnat, M., 2015. Developing pressures: fluid forces driving morphogenesis. Curr Opin Genet Dev 32, 24-30.
Nixon, S.J., Carter, A., Wegner, J., Ferguson, C., Floetenmeyer, M., Riches, J., Key, B., Westerfield, M., Parton, R.G., 2007. Caveolin-1 is required for lateral line neuromast and notochord development. J Cell Sci 120, 2151-2161.
Norman, J., Sorrell, E.L., Hu, Y., Siripurapu, V., Garcia, J., Bagwell, J., Charbonneau, P., Lubkin, S.R., Bagnat, M., 2018. Tissue self-organization underlies morphogenesis of the notochord. Philos Trans R Soc Lond B Biol Sci 373.
Wopat, S., Bagwell, J., Sumigray, K.D., Dickson, A.L., Huitema, L.F.A., Poss, K.D., Schulte-Merker, S., Bagnat, M., 2018. Spine Patterning Is Guided by Segmentation of the Notochord Sheath. Cell Rep 22, 2026-2038.
This is a very lively report of exciting work on the notochord in live zebrafish embryos. The notochords is indeed the hydrostatic spine of the young vertebrate. Congratulations with these interesting findings.
A remark I would like to leave behind is that in human scoliosis, starting at the age of 10 or so, the notochord is no longer present (unless you would call the array of nuclei pulposi in the respective intervertebral discs the notochord). Humans and mammals have intervertebral discs which are not present in other vertebrates. So given the different anatomy and timing of scoliotic deformity, I would hesitate to see zebrafish scoliosis as a proper model for human scoliosis.
This is not to say that the observations are not intriguing and inspiring. Thanks for sharing.
Hi Theo! Thank you for your insights. We do see vacuolated cells remaining in the zebrafish intervertebral disk as well as in the mammalian IVD (pigs and mice). We don’t yet know their function at later stages in the IVD. Our data suggests these cells play a role during spine morphogenesis which is relevant for congenital scoliosis. Adolescent idiopathic scoliosis (AIS), as you suggested, occurs later, involves other tissues, and is not well understood. Thank you for pointing this out! I should have clarified this in the article.