How mechanical signals orchestrate stem cell fate
Posted by Laralynne, on 23 August 2016
Controlling differentiation using biophysical cues from development
Embryonic stem cells have the potential to become any cell type in the adult organism, but coaxing them to a specific fate continues to be a challenge for researchers. While many of the soluble signals involved in patterning the early embryo are well-established, only recently have tools been available to study how biophysical cues synchronize with other signals in the microenvironment to specify cell fate during development. Mechanical signals have been implicated in controlling stem cell fate in a number of contexts, but the molecular mechanisms involved are often not well-defined. By taking cues from embryonic development, we have identified a key role for mechanical signals in driving differentiation of human embryonic stem cells (hESCs) toward mesoderm, work that was recently published in Cell Stem Cell (Przybyla et al., 2016a). We used genetic and pharmacologic manipulation to identify molecular mechanisms whereby tissue tension directs stem cell fate and demonstrated how hESC differentiation protocols can be optimized to direct early progenitor specification.
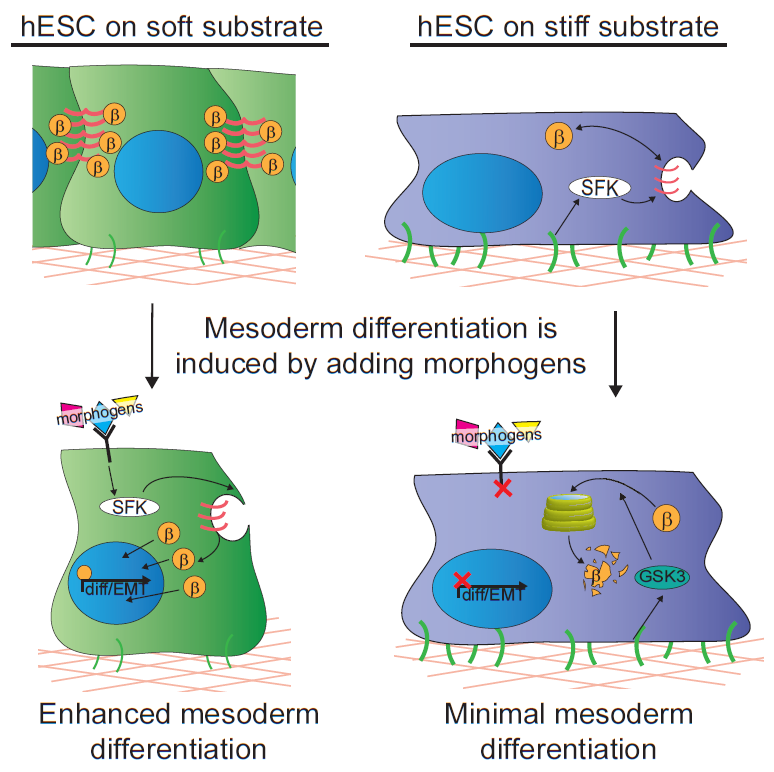
Whether scientists are working to regenerate damaged organs or fix genetic defects in existing organs, a major bottleneck in regenerative medicine is generating differentiated cells that exhibit a structure/function that faithfully recapitulates that of the cells within the tissues in healthy adult organs. One approach has been to mimic the soluble cues and transcriptional signaling cells receive and integrate during embryonic development as they transition to tissue-specific differentiation in the adult organism. Yet cells in tissue also receive spatial-mechanical cues that are critical for directing their fate and maintaining the tissue function.
For more than a decade the Weaver laboratory has been exploring the role of biophysical cues in adult tissue development and homeostasis and malignancy, in order to clarify how the physical context of a cell in a tissue drives embryogenesis and influences stem cell fate specification. To this end, our experimental efforts have focused on examining how cell-cell and cell-extracellular matrix interactions and tissue geometry regulate early developmental decisions. We have been studying early gastrulation and mesoderm specification using human embryonic stem cells and a simplified polyacrylamide gel system combined with three dimensional cell printers. Our findings revealed that the biophysical microenvironment helps to synchronize embryogenesis to facilitate mesoderm progenitor differentiation.
The complexity of embryonic patterning
The first symmetry-breaking process in embryogenesis is gastrulation, when cells are reorganized and divided up into three germ layers that go on to derive all the structures and organs of the body. This process also marks the first epithelial-mesenchymal transition (EMT), as cells in the epiblast ingress into the newly formed primitive streak and lose their epithelial characteristics to form the mesoderm layer. A major question remains as to how cells know where to go and when, and what to do when they get there. Cells derive this information from the inputs they receive from their cellular and noncellular microenvironment. A major goal of our work is to begin to unravel the molecular mechanisms whereby spatial-mechanical cues regulate gastrulation.
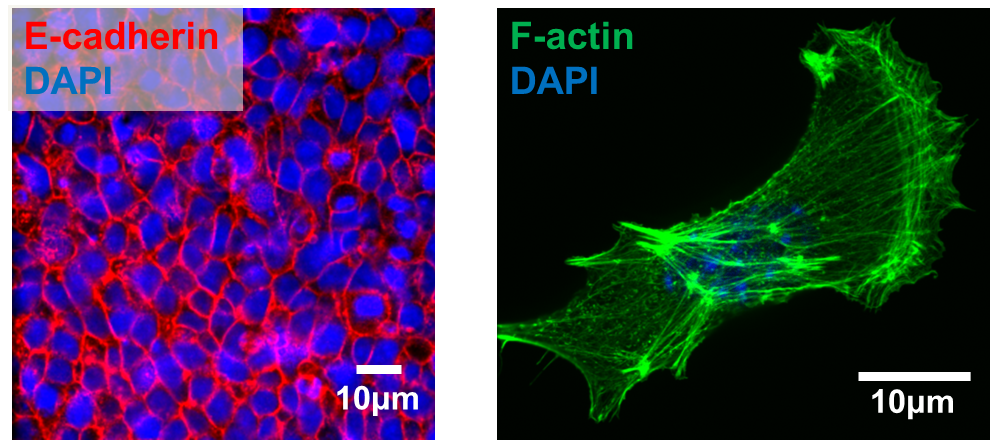
When cells switch between epithelial and mesenchymal states, their organization with respect to each other becomes dramatically altered. Cells in an epithelial state have strong cell-cell contacts and exhibit collective cell behaviors such as coordinated migration and mechanical connectivity, whereas cells in a mesenchymal state acquire a motile phenotype that is linked in part to stronger cell-extracellular matrix interactions. After the initial EMT during gastrulation that forms the mesoderm layer, mesoderm cells destined to different downstream fates undergo additional mesenchymal-epithelial transitions (METs) and EMTs as tissues are formed and cells migrate to their appropriate embryonic positions. The objective of our recent studies was to clarify how cell arrangements and rearrangements influenced the cellular response to mesoderm-inducing cues in the context of early embryonic gastrulation. To do this, we first developed a method to control both the hESC plating geometry and density and the mechanical signals presented to hESCs through their extracellular matrix substrate (Lakins et al., 2012; Przybyla et al., 2015). We then used this system to interrogate how cell context influences early mesoderm specification and is able to orchestrate a program of differentiation with striking parallels to embryonic gastrulation.
Mechanical signals can control transcriptional responses
The mechanical stimuli sensed by cells in the developing embryo include the stiffness and topography of their extracellular matrix, the forces exerted between cells, the shear stress applied by surrounding fluid flow, and mechanical strains resulting from interstitial and osmotic pressures. Cells respond to mechanical signals with integrated mechanosensitive machinery, including molecules that hold tension at the cell-cell and cell-substrate interfaces. Cell organization within tissues affects what mechanosensitive machinery is expressed and activated, thereby dictating how cells respond to other environmental cues (Przybyla et al., 2016b). For example, mesenchymal cells may initiate signaling pathways downstream of strong cell-matrix contacts at focal adhesions, whereas strong cell-cell junctions in epithelial cells may allow for efficient transmission of contact-based signals. Mechanical signals can also alter ligand (Giacomini et al., 2012; Wipff et al., 2007) or receptor (Boulant et al., 2011; Gauthier et al., 2011) accessibility, thereby enhancing or inhibiting downstream signaling pathways.
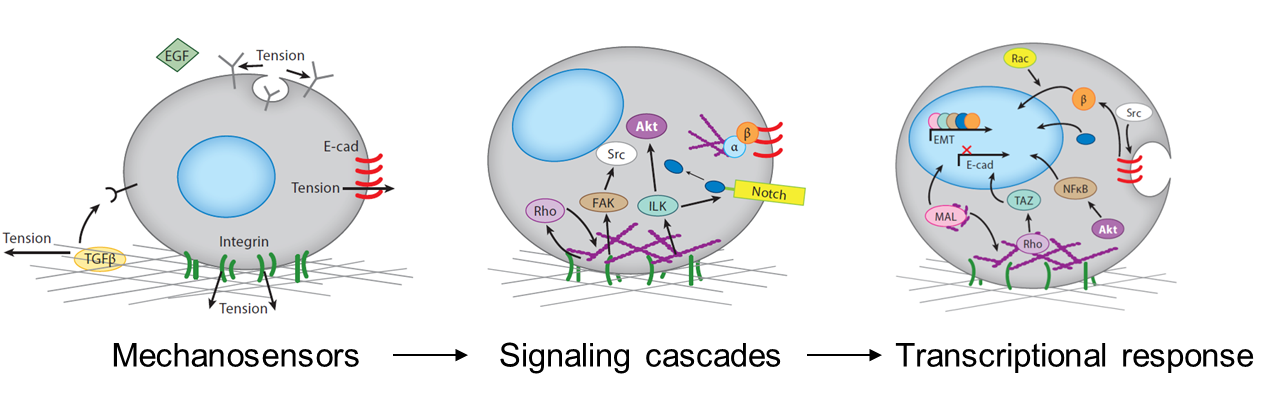
Tissues and organs all have an intrinsic stiffness that is likely to affect their functionality. When tissue stiffness is altered, as in the case of heart or liver fibrosis, the ability of the organ to function is dramatically compromised. Similarly, developing embryonic tissues have an intrinsic stiffness that rapidly changes during differentiation (Majkut et al., 2013). Though we believe that these intrinsic properties contribute to development as part of the signaling microenvironment that drives cell fate, this has not been demonstrated in a controlled way. Using engineered matrices to control substrate stiffness as a mechanical parameter allowed us to explore this phenomenon during stem cell differentiation in vitro, and relate this to processes that occur in vivo during embryonic development.
Stem cell fate can be controlled by substrate stiffness as a mechanical input
In 2006, Dennis Discher’s group reported that mesenchymal stem cell fate could be controlled by altering substrate stiffness (Engler et al., 2006). Since then, many studies have demonstrated that substrate compliance is able to influence mesenchymal, neuronal, and liver stem cell differentiation (Cozzolino et al., 2016; Gobaa et al., 2015; Saha et al., 2008) as well as stem cell self-renewal (Chowdhury et al., 2010; Gilbert et al., 2010) and they have implicated RhoGTPases and myosins as key regulators of these behaviors. Nevertheless, the relevance of these observations to human embryonic stem cell fate and the molecular mechanisms critical for eliciting these phenotypes remained ill defined.
In our study, we cultured human embryonic stem cells on soft substrates similar in compliance to a gastrulation-stage embryo (400Pa) and substrates that were stiffer by two orders of magnitude. We were able to show that hESCs maintain their ability to grow and self-renew regardless of substrate stiffness, but surprisingly we failed to observe any direct impact of substrate stiffness on hESC differentiation. Nevertheless, we did note a remarkable increase in their propensity to differentiate into mesoderm progenitors when they were stimulated with differentiation-promoting morphogens if grown on the extracellular substrates that mimicked the microenvironment of the embryo. Mesoderm differentiation occurs concomitant with the first EMT of development, so we concluded that it could represent a stage at which cells respond to mechanical inputs by altering their fate.
We next explored the molecular mechanisms underlying the enhanced mesoderm progenitor specification, finding that hESCs on embryo-like substrates coordinated several complementary pathways to initiate and stabilize mesoderm progenitor differentiation. First, β-catenin was not degraded in cells cultured on soft substrates, while rapid proteasomal degradation occurred in cells on stiff substrates due to increased Src and GSK3 activity. Second, cell-cell junctions were stabilized in cells on soft but not stiff substrates, creating colonies with enhanced epithelial characteristics, including circumferential actin and high levels of E-cadherin and β-catenin localized at these junctions. Third, the induction of differentiation caused controlled activation of signals, including P120-catenin-mediated Kaiso sequestration, that coordinately enhanced the ability of β-catenin to enter the nucleus and begin a program of transcription to initiate and reinforce mesoderm differentiation. By upregulating Wnts, this system propagated a feed-forward loop that mitigated expression of secreted Wnt inhibitors to ensure robust, efficient mesoderm differentiation, but only under circumstances where cells were primed on soft, embryo-like substrates. Our findings obtained using this simplified hESC system not only build on prior knowledge of Wnt-dependent mesoderm specification during embryogenesis derived from model organisms, but also definitively link Wnt-stimulated developmental programs to a tightly coordinated cytoskeletal-linked adhesion-directed mechanosignaling pathway.
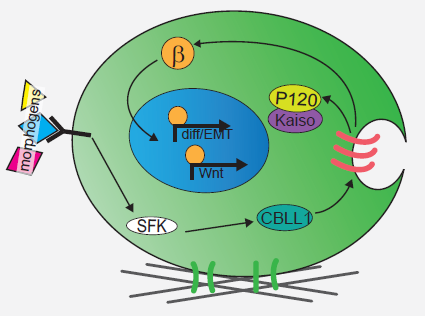
Using an in vivo model of gastrulation, the chick embryo, we also demonstrated that some of the same signaling pathways were upregulated in cells undergoing gastrulation and differentiating toward mesoderm. This study therefore demonstrates a means by which soluble signals synchronize with mechanical cues to drive hESC fate decisions, implying that biophysical parameters sensed by cells in vivo are an important part of the extracellular microenvironment that contributes to cell specification during development.
Understanding the cues required for differentiation during development is a crucial step towards tissue engineering-based cell therapies
Stem cell-based regenerative therapies require generation of specific cell types that perform their physiological function and are arranged properly within tissues. Pluripotent stem cells are particularly important in regenerative medicine because patient-specific induced pluripotent stem cells (iPSCs) can be derived and used to fix genetic defects or regenerate diseased or injured organs without risk of immune rejection. Although protocols exist to differentiate pluripotent cells into specific cell types in vitro, many such protocols generate immature cells in which tissue-specific marker expression is much lower than levels found in corresponding primary adult cell cultures, and these cells often lack the ability to adequately perform the functions required in fully functional tissues.
By demonstrating how one aspect of the biophysical environment, substrate compliance, can be tuned to influence embryonic stem cell fate in a developmentally relevant way, we have taken a step towards recapitulating developmental processes in the dish, and we plan to extend these findings to explore how mesoderm cells incorporate biophysical cues to further differentiate toward a cardiac fate. We hope that recapitulating more aspects of the biophysical microenvironment of the embryo, including shear stresses and mechanical strains, will allow us to develop differentiation protocols that result in functional cells that will be useful in regenerative therapies. To this end, our objective is to further characterize the mechanical properties of the early embryo and develop additional innovative techniques to recapitulate the in vivo embryonic microenvironment in a dish.
References
Boulant, S., Kural, C., Zeeh, J.-C., Ubelmann, F., and Kirchhausen, T. (2011). Actin dynamics counteract membrane tension during clathrin-mediated endocytosis. Nat. Cell Biol. 13, 1124–1131.
Chowdhury, F., Li, Y., Poh, Y.-C., Yokohama-Tamaki, T., Wang, N., and Tanaka, T.S. (2010). Soft Substrates Promote Homogeneous Self-Renewal of Embryonic Stem Cells via Downregulating Cell-Matrix Tractions. PLoS ONE 5, e15655.
Cozzolino, A.M., Noce, V., Battistelli, C., Marchetti, A., Grassi, G., Cicchini, C., Tripodi, M., and Amicone, L. (2016). Modulating the Substrate Stiffness to Manipulate Differentiation of Resident Liver Stem Cells and to Improve the Differentiation State of Hepatocytes. Stem Cells Int. 2016, 5481493.
Engler, A.J., Sen, S., Sweeney, H.L., and Discher, D.E. (2006). Matrix Elasticity Directs Stem Cell Lineage Specification. Cell 126, 677–689.
Gauthier, N.C., Fardin, M.A., Roca-Cusachs, P., and Sheetz, M.P. (2011). Temporary increase in plasma membrane tension coordinates the activation of exocytosis and contraction during cell spreading. Proc. Natl. Acad. Sci. U. S. A. 108, 14467–14472.
Giacomini, M.M., Travis, M.A., Kudo, M., and Sheppard, D. (2012). Epithelial cells utilize cortical actin/myosin to activate latent TGF-β through integrin α(v)β(6)-dependent physical force. Exp. Cell Res. 318, 716–722.
Gilbert, P.M., Havenstrite, K.L., Magnusson, K.E.G., Sacco, A., Leonardi, N.A., Kraft, P., Nguyen, N.K., Thrun, S., Lutolf, M.P., and Blau, H.M. (2010). Substrate elasticity regulates skeletal muscle stem cell self-renewal in culture. Science 329, 1078–1081.
Gobaa, S., Hoehnel, S., and Lutolf, M.P. (2015). Substrate elasticity modulates the responsiveness of mesenchymal stem cells to commitment cues. Integr. Biol. Quant. Biosci. Nano Macro 7, 1135–1142.
Lakins, J.N., Chin, A.R., and Weaver, V.M. (2012). Exploring the link between human embryonic stem cell organization and fate using tension-calibrated extracellular matrix functionalized polyacrylamide gels. Methods Mol. Biol. Clifton NJ 916, 317–350.
Majkut, S., Idema, T., Swift, J., Krieger, C., Liu, A., and Discher, D.E. (2013). Heart-specific stiffening in early embryos parallels matrix and myosin expression to optimize beating. Curr. Biol. CB 23, 2434–2439.
Przybyla, L., Lakins, J.N., Sunyer, R., Trepat, X., and Weaver, V.M. (2015). Monitoring developmental force distributions in reconstituted embryonic epithelia. Methods 94, 101-113.
Przybyla, L., Lakins, J.N., and Weaver, V.M. (2016a). Tissue Mechanics Orchestrate Wnt-Dependent Human Embryonic Stem Cell Differentiation. Cell Stem Cell, in press.
Przybyla, L., Muncie, J.M., and Weaver, V.M. (2016b). Mechanical Control of Epithelial-to-Mesenchymal Transitions in Development and Cancer. Annu. Rev. Cell Dev. Biol. 32, in press.
Saha, K., Keung, A.J., Irwin, E.F., Li, Y., Little, L., Schaffer, D.V., and Healy, K.E. (2008). Substrate modulus directs neural stem cell behavior. Biophys. J. 95, 4426–4438.
Wipff, P.-J., Rifkin, D.B., Meister, J.-J., and Hinz, B. (2007). Myofibroblast contraction activates latent TGF-beta1 from the extracellular matrix. J. Cell Biol. 179, 1311–1323.