Secret talk between epithelium and endothelium determines hair follicle stem cell fate
Posted by PrachiJain, on 6 November 2019
By Kefei Nina Li and Prachi Jain
Stem cells are typically defined by their ability to self-renew and differentiate. These activities are tightly controlled by both intrinsic cues and extrinsic cues from the microenvironment, known as the SC niche. This niche consists of multiple components, among which blood vessels (BVs) are critical as they not only supply oxygen and nutrients to the SCs but also provide molecular signals. BVs form a perivascular-niche for many adult SCs including neural, mesenchymal and hematopoietic SCs. A molecular connection between SCs and vasculature contributes to tissue homeostasis and repair. However, it remains unclear whether this connection also exists in epithelial stem cells, and it’s also unknown whether SCs can conversely promote remodeling of their own environment for proper tissue homeostasis.
The Tumbar lab at Cornell University uses the mouse hair follicle as a model system to study SCs. Hair follicles (HFs) are characterized by a cyclic destruction and reconstruction, which consists of three morphologically distinct and synchronous phases (Figure 1) : 1) growth and proliferation which results into the formation of a new hair shaft known as anagen; 2) apoptosis driven regression, or catagen; 3) and the resting phase, or telogen. In these three phases, SCs exhibits distinct behaviors such as proliferation, migration, or quiescence.
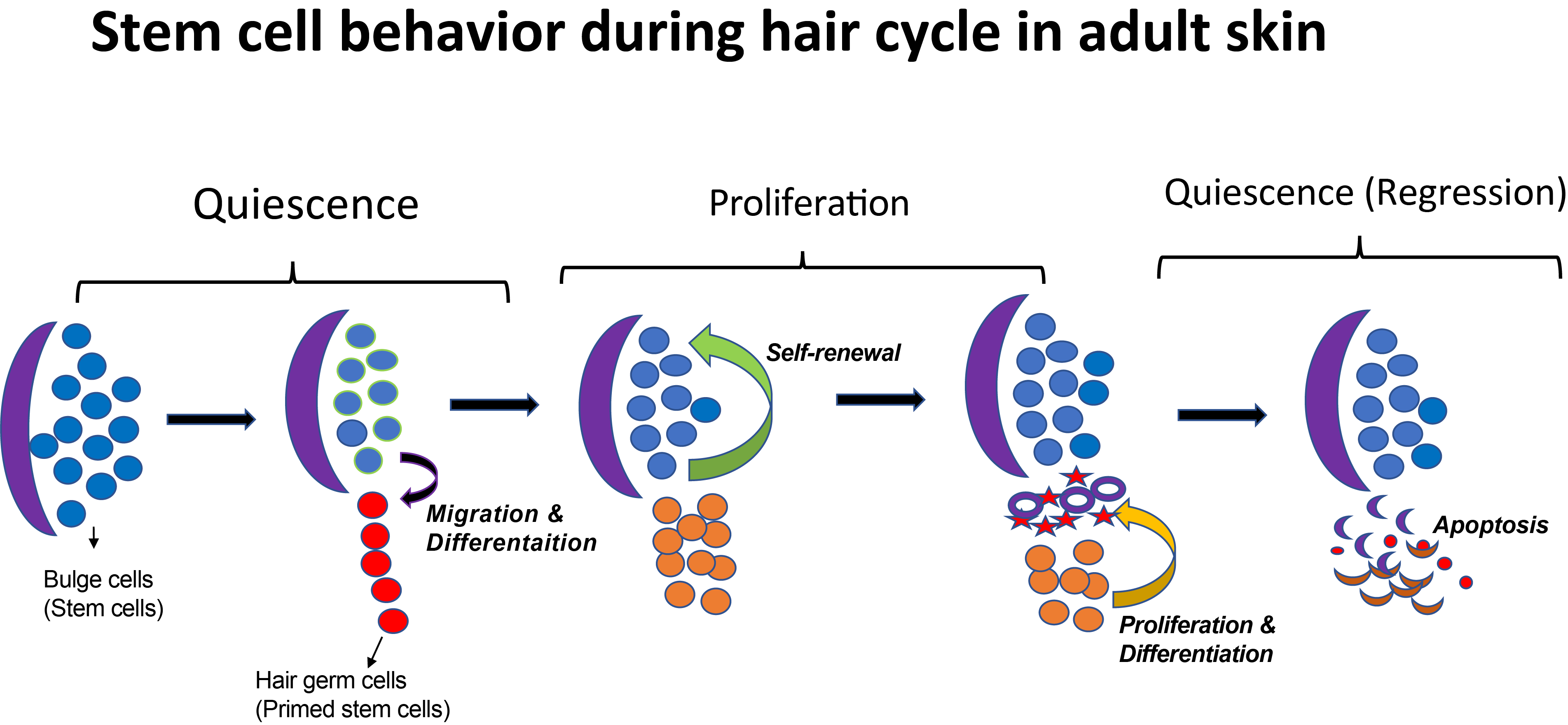
A decade ago, when Tudorita (Doina) was a postdoc with Elaine Fuchs studied the transcriptional profile of the hair follicle stem cells (HFSCs) by purifying label-retaining cells in the lineage tracing experiment (Tumbar et al., 2004). Interestingly, many differentially expressed genes in this population encode secreted molecules, suggesting that in addition to receiving signals from the niche, HFSCs may also modulate the niche (Fuchs et al., 2004). More recently, the Tumbar lab has identified runt-related transcription factor 1 (Runx1) as a HFSC regulator. Epithelial Runx1 knockout mice have significant delay in hair growth (Hoi et al., 2010; Osorio et al., 2008). Runx1 is highly expressed in activated SCs or early progenitor cells and its expression is lost when these cells are proliferating (Figure 1). Microarray analysis further revealed gene expression changes in response to altered Runx1 level in the epithelium (Lee et al., 2014).
Our journey started when Prachi, Post-doc in the lab, got intrigued by the microarray data: the gene changes include secreted molecules whose functions are implicated in vascular remodeling, indicating a cross talk between vasculature and stem cell activation. We started to look for blood vessel remodeling in response to varying levels of Runx1 using mutants: Runx1 epithelial knockout mice (Runx1 EpiKO) and Runx1 epithelial transgenic overexpression mice (Runx1 EpiTG). Blood vessel connection in the skin has mainly been studied for oxygen and nutrition, and its molecular signaling aspect has been addressed in depth. Quick experiments of immunostaining for CD31, an endothelial marker, showed visual differences that were exciting and prompted us to begin our investigation on the connection between HFSC and the vascular niche. We were interested in testing whether the vasculature signals for HFSC activation and/or if HFSCs themselves send signals to remodel vasculature during normal hair homeostasis.
Our basic idea was to perturb one compartment, either epithelium or endothelium, and see what would happen to the other compartment. Taking lead from our earlier observation that BVs are remodeled in response to varying levels of Runx1, undergrad student Catherine focused on quantifying the direct contact between vasculature and different regions of the hair follicle. By subsequent quantification, we found that Runx1 mutants showed distinct patterns of vasculature contact. However, these patterns were at first confusing, and we were not sure how to interpret them.
We then began our analysis by quantifying the area covered by CD31 immunostained vasculature under the hair germ and above the muscle. The quantification process was not easy. First, the selection of CD31 immunostained vasculature needs to be manual, because software such as ImageJ or ilastik are not yet as smart as humans in accurately picking out the vasculature. Second, some of the images were not good quality – for example, some stainings appeared hazy, or in some slides the hypodermis was washed away. Those images could be used for contact quantification, but would not be good for area quantification. Therefore, a lot of stainings had to be repeated. Another problem we encountered the different way different researchers quantified their data. The first part of quantification was done by Flora Eun, a brilliant undergrad who soon graduated and left the lab, and the second part of the job was then picked up by graduate student Nina Li. However, Nina was able to be more precise than Flora in selecting the vasculature, so it did not make sense to combine their data as it would create large error within the dataset. To avoid this problem, Nina then quantified the entire dataset, and compared her statistical result with Flora’s preliminary result. Since both statistical results show the same trend that Runx1 EpiKO has significantly more vasculature than control mice, we felt comfortable to further pursue the study.
We then noticed that the thickness of the hypodermis was different in each mouse, but were able to confirm that this thickness did not relate to the amount of vasculature. Since we reasoned that Runx1 might modulate the vascular niche via secreted molecules, the vasculature in close vicinity to the hair germ should be the most affected population, and they should also be the most important if they can send signals back to the hair germ. We used a more stringent method to quantify vascular differences: we drew a thin stripe under the hair germ, and only quantified the vasculature in this selected region. As expected, Runx1 EpiKO had significantly higher amount of vasculature than control mice, suggesting that HFSCs may actively modulate their own vascular niche via Runx1 expression.
In the reverse signaling, from endothelium to epithelium, we did an initial screen before pursuing a particular mutant. From Dr. Anne Eichmann, we acquired two vasculature-related mutants: Cdh5-CreERT2 mediated endothelial knockout mice for Neuropilin 1 (Nrp1) and activin-receptor like kinase 1 (Alk1) genes (Nrp1 EndKO and Alk1 EndKO). Both genes are critical for endothelial cell homeostasis, and perturbation of either one can lead to serious vasculature related diseases. Since we wanted to know whether perturbation of the endothelium affects hair follicle homeostasis, the idea was to perturb BV during quiescence stage before beginning of next stages of HFSCs proliferation and differentiation. When Prachi started optimizing time points and dosage for knockouts she wasn’t sure that if Alk1 conditional knockout out would be viable and show phenotypes, as previously reported knockout pups could only survive 48 hours after birth. Another question was whether adult vasculature patterns can be remodeled by introducing genetic mutations.
We first checked the hair cycle progression on a few mutant mice after knockout induction at PD17, and sacrificed around PD35. While Nrp1 EndKO did not exhibit obvious epithelial phenotype, we were excited to find Alk1 EndKO showed a hair cycle delay phenotype. Alk1 EndKO in the quiescence stage of hair cycle seemed to modulate vascular remodeling and result in delayed progression of stem cell activation. Therefore, we decided to make further investigation on Alk1 EndKO. To confirm the hair cycle delay phenotype, we checked more mice and at various time points between PD22 to PD35. To distinguish between quiescence and early proliferation, since overall morphology does not differ much at these stages, we did both immunofluorescence staining for Ki67, a proliferation marker, and H&E staining to carefully determine the hair cycle stages for our samples. After checking more than 20 mice, we concluded that Alk1 EndKO mice have delayed proliferation marked by the lack of Ki67 staining. We also confirmed that observed delay was not due to less number of early progenitor cells in the mutant mice but rather as a result of delay in HFSC proliferation.
We then wondered what changes in the skin vasculature could lead to the Alk1 EndKO hair cycle delay phenotype. Again, we did immunofluorescence staining for CD31 and we found that there are more CD31+ vasculature in vicinity of the hair germ. The similarity between Alk1 EndKO and Runx1 EpiKO led us to wonder what is the importance of the vasculature near the hair germ. To answer this question, we first investigated the nature of this vasculature during hair homeostasis using wildtype mice (Figure 2).
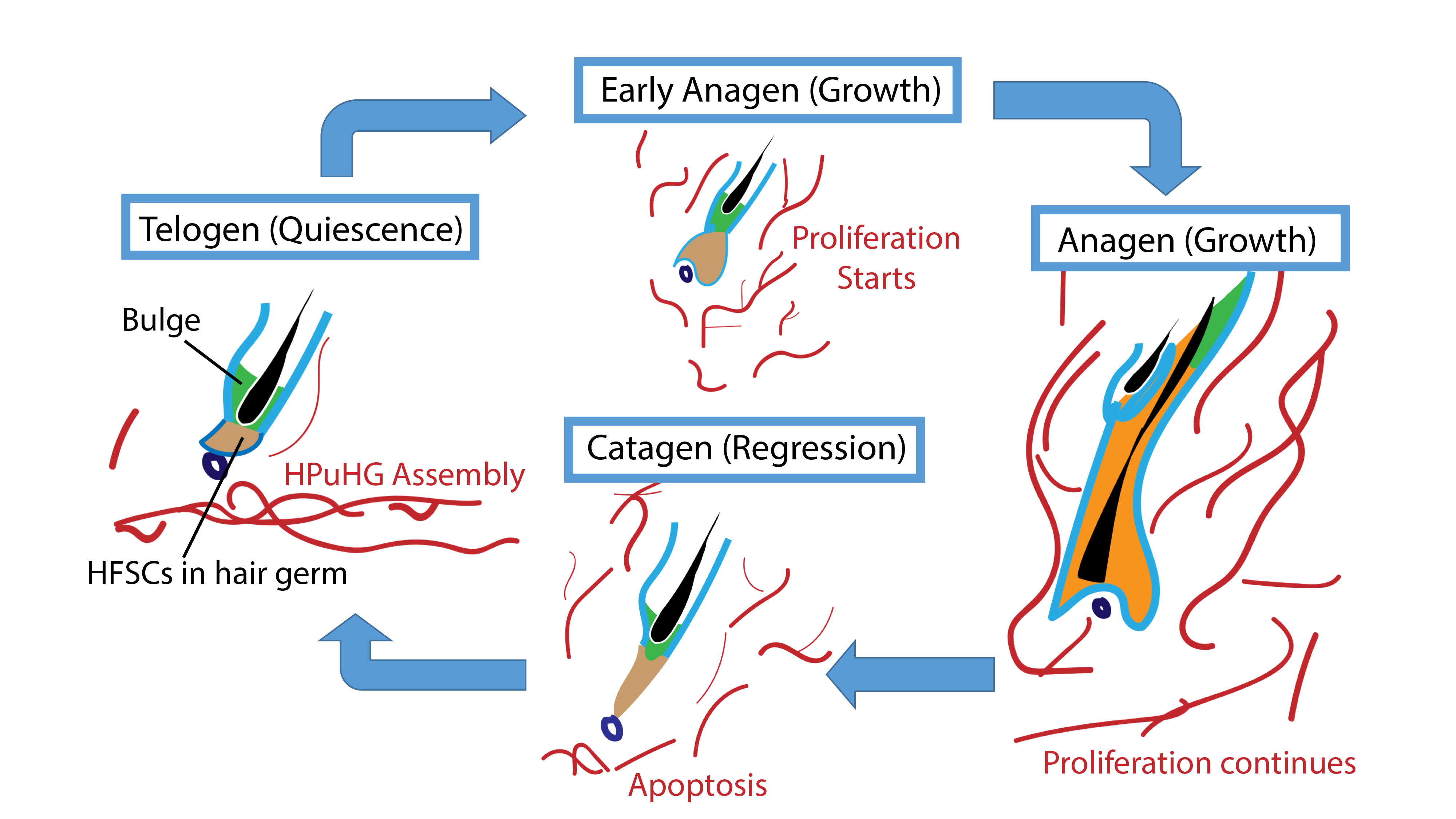
We checked the vasculature arrangement at different stages of the hair cycle. Interestingly, we found that at quiescence stage, a horizontal vascular plexus is formed under the hair germ, which we named it “Horizontal Plexus under Hair Germ (HPuHG)”. This vascular plexus quickly disperses as the hair follicle starts proliferating. Then after apoptosis, the skin vasculature deposits to form the HPuHG again. Since we looked at the quiescence stage in Runx1 EpiKO and in Alk1 EndKO, a stage when HPuHG exists, we believed that the vascular phenotype in the two mutants is in fact an increase in the HPuHG vasculature. These observations strengthened our idea that BVs have distinct role in molecular signaling in addition to nutrition and oxygen, contrary to our belief that increased vasculature causes delay in hair growth. Moreover, distinct pattern of BVs during hair growth supports the idea that BVs are important part of HFSCs niche.
To understand whether molecular signal derived from endothelial cells could cause the HFSC activation defect, we checked if a well-established HFSC quiescence factor BMP4 is also expressed in skin endothelial cells. Previous study indicated endothelial cells express BMP4 in the lung (Frank David et al., 2005). We did immunofluorescence staining for CD31 and BMP4, and found colocalization between the two signals, indicating skin endothelial cells are a source of BMP4. While the expression level of BMP4 in endothelial cells and the average BMP4 intensity in interfollicular epidermis are not altered by the mutation of Alk1 or Runx1, the average intensity of BMP4 in the selected HPuHG region is higher in mutants than in control mice. Western blots also showed elevated BMP4 level in Alk1 EndKO. Though more solid evidence such as RNA-Seq of hair follicle cells from the mutant skin is needed to conclude that endothelial BMP4 is the cause of the HFSC activation delay, our data showed a correlation between BMP4 level, amount of vasculature, and HFSC activation defect. Specifically, our data suggested that excessive HPuHG vasculature may lead to excessive BMP4 in the vicinity of the hair germ, thus delaying the HFSC activation.
There have been previous investigations into the association between the skin vasculature and the hair follicle, but these have focused mainly on hair growth related to vasculature and failed to provide molecular evidence on how the vasculature regulates hair homeostasis (Ellis and Moretti, 1959; Mecklenburg et al., 2000). Our current research focuses on the quiescence stage that has been overlooked for many years, and how cross talk between HFSC and their environment influences their activity. Our data support a model where HFSCs are capable of sending signals to their vascular niche, and the vascular niche can reciprocally control HFSC activation (model summarized in Figure 3).
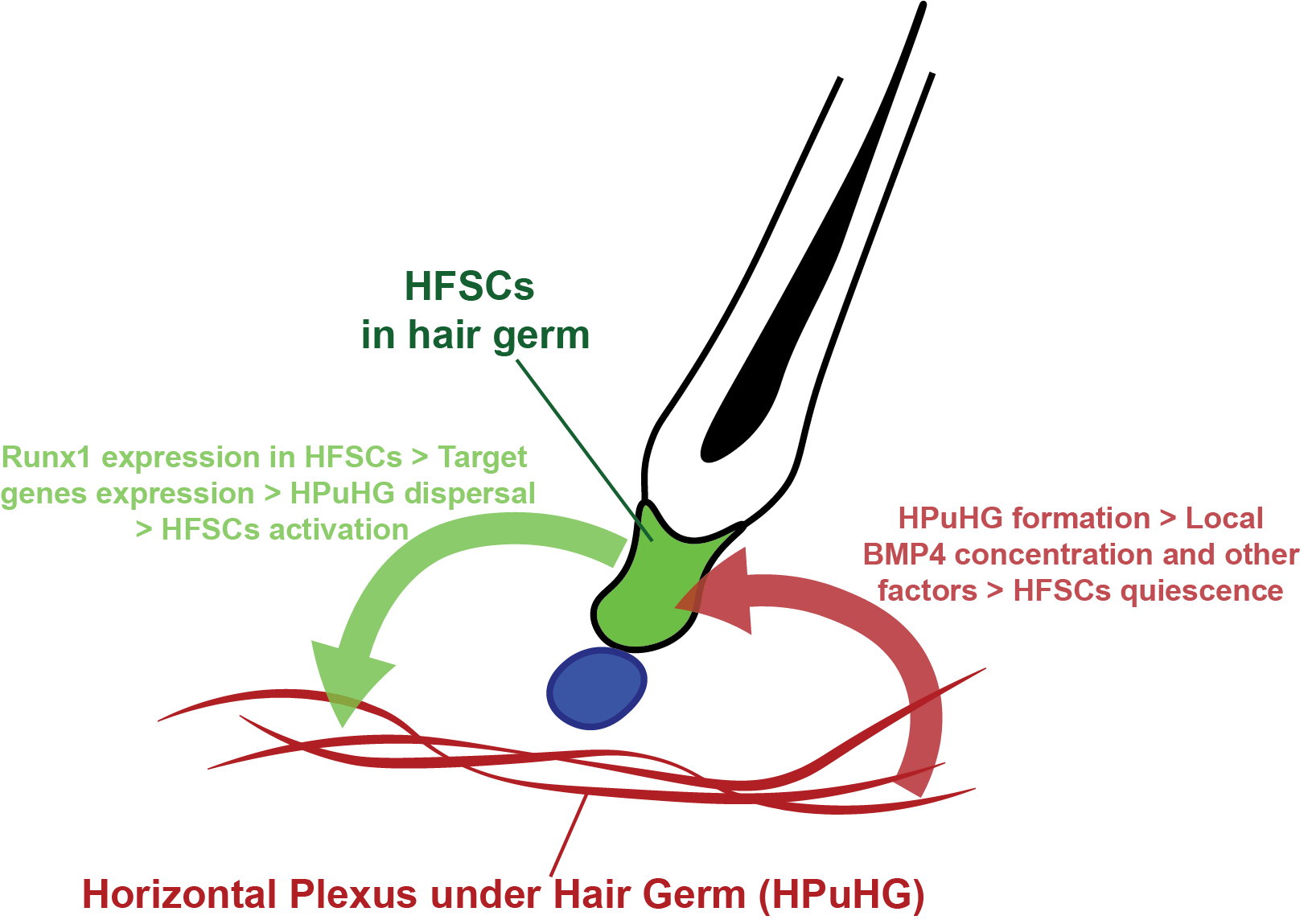
To our knowledge, this is the first publication suggesting a niche modulation role of HFSCs, and also a first documentation of the non-cell autonomous role of Runx1. Though more molecular details underlying the cross-talk between HFSCs and their vascular niche are to be elucidated, our research has opened a new window for future investigation. The Alk1 mutation has been studied in hereditary hemorrhagic telangiectasia, a disease marked by the fusion between arteries and veins. Our current study may further the understanding of its prevalence in skin, and may also provide new thoughts for future therapy. It also has implications for tissue regeneration and in clinical settings where targeting cancer SC niche through anti-angiogenic targets can be promising therapeutics.
Read full story:
Li KN*, Jain P*, He CH, Eun FC, Kang S, Tumbar T.
* Equal contributions
Elife. 2019 Jul 25;8. pii: e45977. doi: 10.7554/eLife.45977.
References
Ellis, R.A., and Moretti, G. (1959). VASCULAR PATTERNS ASSOCIATED WITH CATAGEN HAIR FOLLICLES IN THE HUMAN SCALP. Annals of the New York Academy of Sciences 83, 448-457.
Frank David, B., Abtahi, A., Yamaguchi, D.J., Manning, S., Shyr, Y., Pozzi, A., Baldwin, H.S., Johnson Joyce, E., and de Caestecker Mark, P. (2005). Bone Morphogenetic Protein 4 Promotes Pulmonary Vascular Remodeling in Hypoxic Pulmonary Hypertension. Circulation Research 97, 496-504.
Fuchs, E., Tumbar, T., and Guasch, G. (2004). Socializing with the Neighbors: Stem Cells and Their Niche. Cell 116, 769-778.
Hoi, C.S.L., Lee, S.E., Lu, S.-Y., McDermitt, D.J., Osorio, K.M., Piskun, C.M., Peters, R.M., Paus, R., and Tumbar, T. (2010). Runx1 directly promotes proliferation of hair follicle stem cells and epithelial tumor formation in mouse skin. Molecular and cellular biology 30, 2518-2536.
Lee, Song E., Sada, A., Zhang, M., McDermitt, David J., Lu, Shu Y., Kemphues, Kenneth J., and Tumbar, T. (2014). High Runx1 Levels Promote a Reversible, More-Differentiated Cell State in Hair-Follicle Stem Cells during Quiescence. Cell Reports 6, 499-513.
Mecklenburg, L., Tobin, D.J., Müller-Röver, S., Handjiski, B., Wendt, G., Peters, E.M.J., Pohl, S., Moll, I., and Paus, R. (2000). Active Hair Growth (Anagen) is Associated with Angiogenesis. Journal of Investigative Dermatology 114, 909-916.
Osorio, K.M., Lee, S.E., McDermitt, D.J., Waghmare, S.K., Zhang, Y.V., Woo, H.N., and Tumbar, T. (2008). Runx1 modulates developmental, but not injury-driven, hair follicle stem cell activation. Development 135, 1059.
Plikus, M.V., Mayer, J.A., de la Cruz, D., Baker, R.E., Maini, P.K., Maxson, R., and Chuong, C.-M. (2008). Cyclic dermal BMP signalling regulates stem cell activation during hair regeneration. Nature 451, 340+.
Tumbar, T., Guasch, G., Greco, V., Blanpain, C., Lowry, W.E., Rendl, M., and Fuchs, E. (2004). Defining the epithelial stem cell niche in skin. Science 303, 359-363.
Zhang, J., He, X.C., Tong, W.-G., Johnson, T., Wiedemann, L.M., Mishina, Y., Feng, J.Q., and Li, L. (2006). Bone Morphogenetic Protein Signaling Inhibits Hair Follicle Anagen Induction by Restricting Epithelial Stem/Progenitor Cell Activation and Expansion. STEM CELLS 24, 2826-2839.