The mechanics of crocodile head scale development
Posted by Michel Milinkovitch, on 11 December 2024
Rory L. Cooper, Ebrahim Jahanbakhsh & Michel C. Milinkovitch
Laboratory of Artificial and Natural Evolution (LANE), Geneva, Switzerland
https://www.lanevol.org
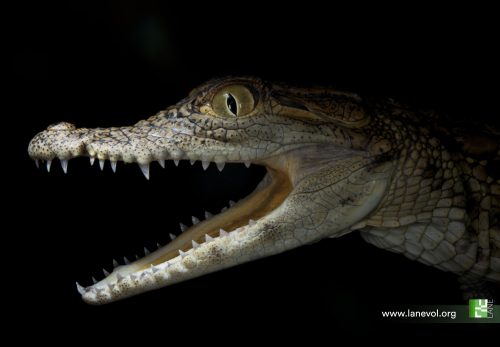
Credits: M. C. Milinkovitch & A. Debry
© michel.Milinkovitch@unige.ch — University of Geneva, Switzerland.
From the hard, protective scales of reptiles to the soft, insulating fur of mammals, amniotes are equipped with a remarkable range of skin appendages that help them thrive in diverse environments. To fulfil their various functions, such as mechanical protection, thermoregulation, and the provision of camouflage, skin appendages must be precisely arranged (or patterned). For instance, birds display a regular spatial arrangement of feathers which enable flight (1), whereas snakes exhibit a near-perfect hexagonal pattern of scales, which are aligned to their muscles and ribs (2), to aid locomotion.
All of these skin appendages develop from ‘placodes’ (3, 4) comprised of an epidermal thickening, an underlying aggregation of dermal cells, and conserved molecular signalling. It is well-established that the spatial patterning of placodes is self-organised through interactions between activatory and inhibitory morphogens, forming a so-called Turing reaction-diffusion system (5-7). In other words, the resulting self-organised “polka-dot” arrangement of gene expression provides a template defining where skin appendages will develop (1, 3, 4, 8).
Remarkably, the irregular polygon-shaped head scales of crocodiles present a fascinating exception to this paradigm, as they appear to emerge from a mechanical process, i.e., in the total absence of a genetic template (9). However, the exact nature of this process has remained elusive for more than a decade. In our new article published this week in Nature (10), we solve this mystery through a multidisciplinary approach combining experimental manipulations of developing crocodile embryos, cutting-edge light sheet microscopy, and state-of-the-art numerical simulations.
First, we imported a large number of Nile crocodile eggs from South Africa to our laboratory in Geneva, Switzerland. After incubating these embryos until their head scales begin to emerge, we performed precise in-ovo intravenous injections of epidermal growth factor (EGF) protein that, as its name indicates, increases epidermal growth while also promoting keratinisation. As we increased the dose of EGF, we observed the development of an extensive labyrinthine pattern of convoluted folds across the jaws of the crocodile embryo, instead of the normal polygonal scales. These results demonstrate that crocodile head scales develop through compressive folding and that EGF treatment exacerbates this folding. Note that tissue buckling from ‘constrained growth’ is thought to shape other biological forms, including the intricate folds of the human brain (11), villification of the human and chicken gut (12), the surface wrinkling of mucosa (13), and the small polygons on the glabrous skin of the rhinarium (or naked nose) of dogs, cows and ferrets (14). Interestingly, we have recently showed that, for this latter example, the polygonal form of the pattern is imposed by the underlying polygonal pattern of blood vessels that act as ‘mechanical positional information’ (14).
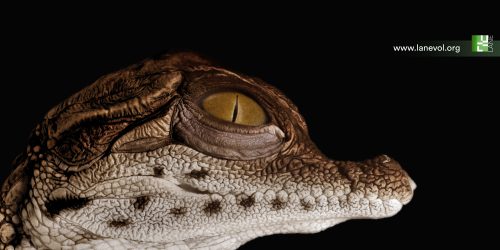
Credits: Rory Cooper & M. C. Milinkovitch
© michel.Milinkovitch@unige.ch — University of Geneva, Switzerland.
Remarkably, when we allow the crocodiles to hatch four weeks after the EGF treatment has finished, they no longer display a fully labyrinthine pattern, as it partially relaxes into a pattern of smaller polygons. Importantly, this pattern resembles that of another crocodilian species – the spectacled caiman. Therefore, simply by promoting skin growth and differentiation during embryonic development, we can alter the hatchling head scale pattern from that of a Nile crocodile to that of a caiman. Therefore, shifts in the molecular components of an intricate Turing reaction-diffusion system are not required to explain the evolution of head scale patterns in crocodilians. Instead, slight variations in the expression of a very small number of genes affecting skin growth and/or stiffness can clearly explain this macroevolutionary change.
We next used cutting-edge light sheet microscopy to build a realistic 3D computer model of the crocodile embryo’s head before the emergence of scales. This model incorporates the key elements that we suggest determine the mechanical patterning of crocodile head scales. This includes the geometry of the skin (such as the spatial variation of epidermal and dermal thicknesses) and underlying bone, the organisation of collagen fibres within the dermis, and the relative growth rates of the two skin layers. Remarkably, our computer growth simulations accurately reproduce the natural head scale arrangement of Nile crocodiles, which includes elongated units on top of the jaws and smaller polygons on the sides. In addition, increasing both skin growth and stiffness in our simulations replicates the labyrinthine brainy folding seen in our EGF-treated embryos. These results show that the polygonal arrangement of crocodile head scales can indeed emerge from a purely mechanical system of constrained skin growth, in the complete absence of self-organising gene interactions.
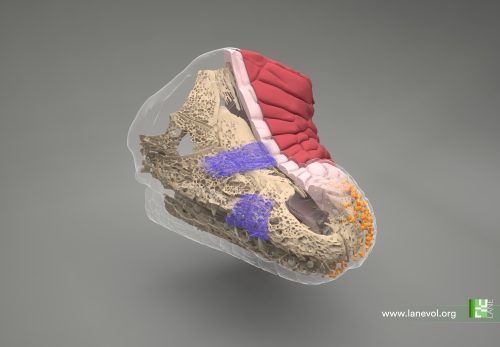
Credits: F. Berger & M. C. Milinkovitch
© michel.Milinkovitch@unige.ch — University of Geneva, Switzerland.
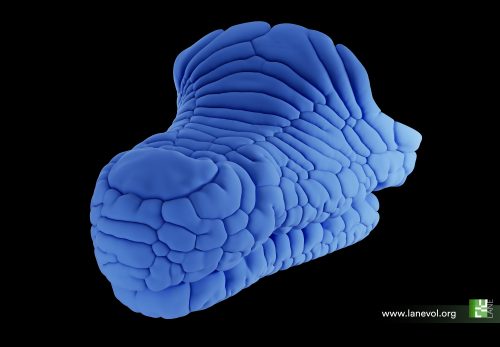
Credits: E. Jahanbakhsh & M. C. Milinkovitch
© michel.Milinkovitch@unige.ch — University of Geneva, Switzerland.
Overall, our findings show that the polygonal head scales of crocodiles emerge from compressive folding of the skin. Importantly, natural variations to the skin’s growth and material properties have allowed the evolution of diverse head scale patterns observed across different crocodilian species. Therefore, while gene interactions can drive many examples of embryonic patterning (7), self-organisation in development is not restricted to such chemical interactions. Indeed, self-organised physical processes also contribute to the emergence of intricate biological forms (15-17).
Check out our (hopefully appealing) 3D imaging data and numerical simulations in the following video summarising our results:
———————————————————————
———————————————————————
———————————————————————
Versions in other languages (Spanish, German, Japanese and Chinese) will be made available in January 2025.
References
1. W. K. W. Ho et al., Feather arrays are patterned by interacting signalling and cell density waves. PLoS biology 17, e3000132 (2019).
2. A. C. Tzika, A. Ullate-Agote, S. Zakany, M. Kummrow, M. C. Milinkovitch, Somitic positional information guides self-organized patterning of snake scales. Sci Adv 9, eadf8834 (2023).
3. N. Di-Poï, M. C. Milinkovitch, The anatomical placode in reptile scale morphogenesis indicates shared ancestry among skin appendages in amniotes. Science Advances 2, e1600708 (2016).
4. R. L. Cooper et al., An ancient Turing-like patterning mechanism regulates skin denticle development in sharks. Science Advances 4, eaau5484 (2018).
5. A. M. Turing, The Chemical Basis of Morphogenesis. Philosophical Transactions of the Royal Society of London. Series B, Biological Sciences 237, 37-72 (1952).
6. S. Kondo, T. Miura, Reaction-Diffusion Model as a Framework of Understanding Biological Pattern Formation. Science (New York, N.Y.) 329, 1616-1620 (2010).
7. M. C. Milinkovitch, E. Jahanbakhsh, S. Zakany, The Unreasonable Effectiveness of Reaction Diffusion in Vertebrate Skin Color Patterning. Annu Rev Cell Dev Biol 39, 145-174 (2023).
8. S. Sick, S. Reinker, J. Timmer, T. Schlake, WNT and DKK determine hair follicle spacing through a reaction-diffusion mechanism. Science 314, 1447-1450 (2006).
9. M. C. Milinkovitch et al., Crocodile Head Scales Are Not Developmental Units But Emerge from Physical Cracking. Science 339, 78-81 (2013).
10. G. N. Santos-Durán, R. L. Cooper, E. Jahanbakhsh, G. M. Timin, Michel. C., Self-organised Patterning of Crocodile Head Scales by Compressive Folding. Nature, 11 December 2024.
11. T. Tallinen et al., On the growth and form of cortical convolutions. Nature Physics 12, 588-593 (2016).
12. A. E. Shyer et al., Villification: How the Gut Gets Its Villi. Science 342, 212-218 (2013).
13. B. Li, Y.-P. Cao, X.-Q. Feng, H. Gao, Surface wrinkling of mucosa induced by volumetric growth: Theory, simulation and experiment. Journal of the Mechanics and Physics of Solids 59, 758-774 (2011).
14. P. Dagenais et al., Mechanical positional information guides the self-organized development of a polygonal network of creases in the skin of mammalian noses. Curr Biol, (2024).
15. B. Li, Y. P. Cao, X. Q. Feng, H. J. Gao, Mechanics of morphological instabilities and surface wrinkling in soft materials: a review. Soft Matter 8, 5728-5745 (2012).
16. A. J. Hughes et al., Engineered Tissue Folding by Mechanical Compaction of the Mesenchyme. Dev Cell 44, 165-178 e166 (2018).
17. A. Bailles, E. W. Gehrels, T. Lecuit, Mechanochemical Principles of Spatial and Temporal Patterns in Cells and Tissues. Annu Rev Cell Dev Biol 38, 321-347 (2022).