Zipping up the neural tube
Posted by Matteo Molè, on 21 April 2020
Matteo A. Molè & Andrew J. Copp
Molè et al., Integrin-Mediated Focal Anchorage Drives Epithelial Zippering during Mouse Neural Tube Closure. Dev. Cell. 52, 321-334.e6 (2020).
Zippering is a striking phenomenon whereby two opposing epithelial tissues become progressively united in one direction over a period of time. Similar, to the travel of a zip fastener, zippering leads to forward progression of a point of fusion, and can occur over significant distances along an organ or tissue, to eventually seal an opening. This is fundamental to establish de novo continuity between two opposing epithelial layers in the embryo, or to repair a gap for example following an injury (Begnaud et al., 2016; Jacinto et al., 2001; Martin and Parkhurst, 2004).
In development, zippering can be first observed during morphogenesis of the neural tube, whereby the flat 3-layered embryo, resulting from gastrulation, undergoes a major transformation to establish the final 3D basic body plan of the future fetus. This remarkable reshaping event is driven by precise coordination between: (i) invagination of the neural plate along the midline, (ii) elevation of the neural folds to meet at the dorsal midline, and (iii) continuous progression of zippering to seal the entire rostro-caudal axis of the developing embryo (reviewed in Nikolopoulou et al., 2017).
Failure of neural tube closure results in the development of severe congenital malformations, collectively known as neural tube defects (NTDs) (Copp et al., 2013; Greene and Copp, 2014; Wallingford et al., 2013). As 0.5 to 2 in every 1000 established pregnancies are affected world-wide, the clinical implications are highly significant to our society. Open spina bifida is the most common NTD observed in new-born babies, caused by failure to complete spinal neural closure by the end of the first month of gestation (Copp et al., 2015).
Alongside the neural tube, several other organs employ epithelial zippering as a mechanism for their development: for example, the optic fissure, palatal shelves, tracheoesophageal foregut and presumptive genitalia. Failure of zippering leaves the organ unsealed, resulting in severe open defects such coloboma, cleft palate, tracheoesophageal fistula and hypospadias.
Our story started with a completely unexpected observation in 2013 which brought us to explore the mechanism behind epithelial zippering during closure of the mouse neural tube. Initially, we aimed to investigate the role of cell-extracellular matrix (ECM) interactions during neural tube closure as a whole. We started examining the structure of the major ECM components including laminins, collagen IV and fibronectin, together with expression of the main integrin subunits that mediate these interactions. However, we noticed that a specific integrin receptor combination, a5b1, localised precisely at the site of zippering. Furthermore, it did not seem a coincidence that this site was also enriched for thick radially-oriented fibrils of fibronectin, which represents the central interacting ligand of a5b1 integrin (Figure 1).
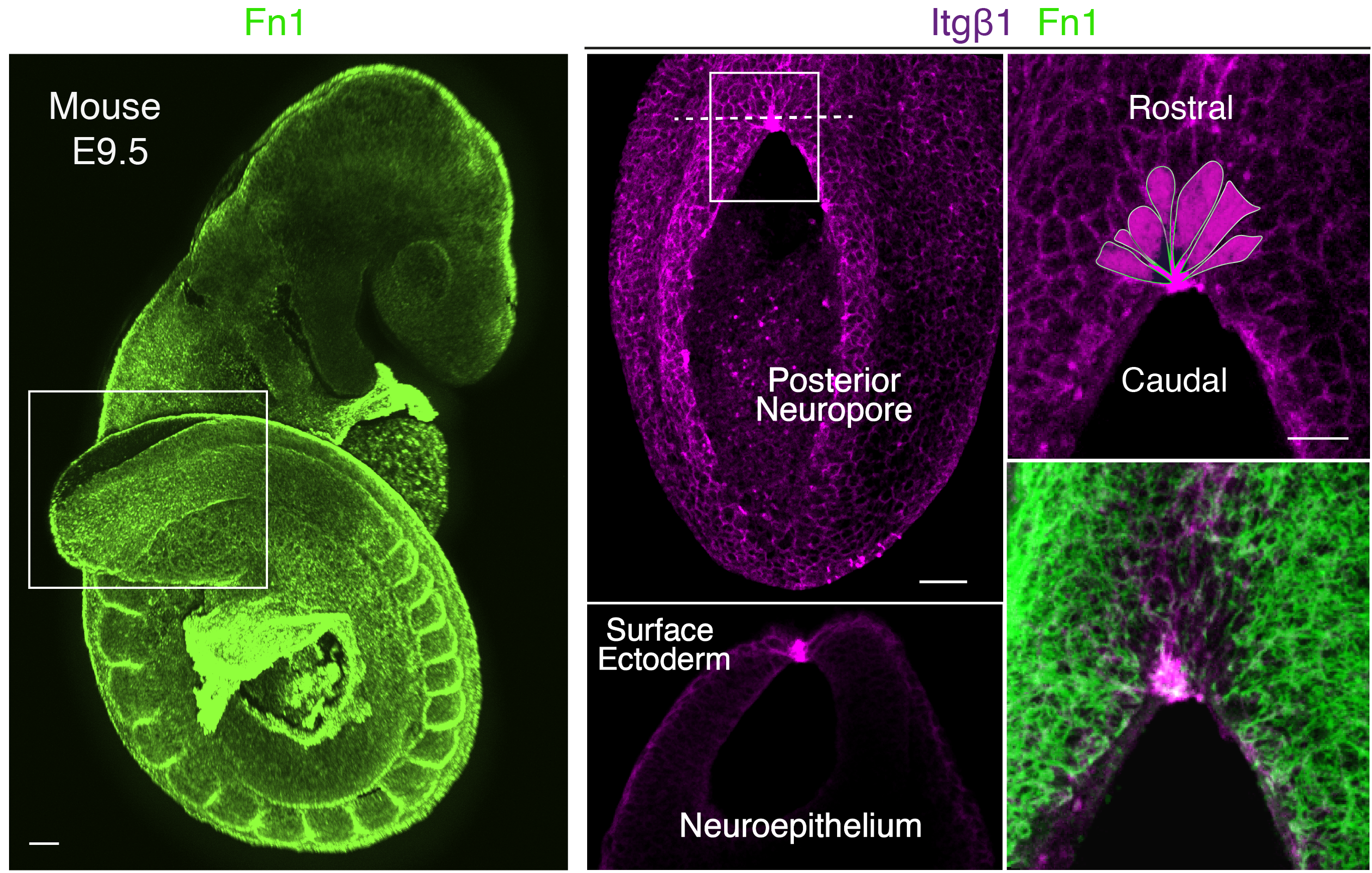
Therefore, we decided to embark on a highly risky and (so it turned out) long experimental route to genetically inactivate the integrin b1 subunit, to assess if the inability to interact with fibronectin at this site would affect propagation of zippering. The integrin b1 knockout mouse dies early in development (Fassler and Meyer, 1995; Stephens et al., 1995) so we had to use conditional genetic approaches. We targeted the neuroepithelium, as the precursor of the neural tube, and we separately targeted the surface ectoderm, that connects to the neuroepithelium at the zippering point. The experiments showed that integrin b1 expression is required on surface ectoderm cells, and its inactivation impaired fusion leading to highly penetrant spina bifida. This discovery was a really exciting and rewarding moment of our research. However, we then had another important question to answer: how do these local cell-ECM interactions, via integrin receptor a5b1, mediate progression of zippering and closure of the neural tube?
It took a long time to find the missing piece of the puzzle to solve the above question. At the beginning, we looked at the cytoskeleton, cellular protrusions, cell death and cell proliferation, which are all downstream of the highly intricate integrin signalling network (Barczyk et al., 2010; Lowell and Mayadas, 2012; Schwartz, 2010). However, we could not find a clear answer by exploring any of these avenues. A very inspiring study was the paper from Edwin Munro’s group (Hashimoto et al., 2015) which involved live imaging of neural tube zippering in the ascidian Ciona intestinalis. The authors described a process of sequential cell junction shortening at the site of zippering. Therefore, we decided to zoom in and look at the cell shapes in the closing mouse neural tube and, similar to Ciona, we noticed that cells around the site of zippering had significantly shorter junctions compared to those that had not yet entered the zippering point. In very elegant geometry, the cells adopted a wedge shaped morphology and formed a semi-rosette configuration which converged precisely to a central fulcrum where the integrins localised (Figure 1). In the targeted mutant embryos, the semi-rosette was severely disrupted which prevented the cell junctions from converging.
At this time, we submitted the paper and received very positive responses. However, we faced a further big challenge: one of the reviewers asked us to live image the process to see if indeed the dynamics of cell shape change and junction remodelling, as predicted by our model, could be observed in vivo. Live imaging of mouse neural tube closure is technically very challenging, as the embryos need to be maintained in culture in a healthy state, with optimal heartbeat, and yet need to be static for confocal imaging. The work from Lee Niswander’s group (Massarwa and Niswander, 2013) had shown that live imaging of mouse embryos is possible, but their images lacked the resolution we needed to visualise individual cell behaviour. We decided to take on the challenge and, after many attempts and long evenings and weekends spent in the confocal room, we finally managed to get images with sufficient cellular resolution. Thanks to the exceptional dissecting skills of Gabriel Galea, we made a small hole through the yolk sac and amnion to expose the closing spinal neural folds, and then kept the embryo steady on an agarose surface using an implanted microsurgical needle. The embryos were very healthy – their hearts beat vigorously – but this meant we had to manually acquire each z-stack and re-centre the region of interest each time!
Despite all the effort, the result was worth it. After a post-imaging surface subtraction, to remove the underlying tissue, we could clearly see for the first time the live behaviour of the cells and how zippering propagates along the body axis. Cells in close proximity to the site of fusion display shorter proximal junctions than cells that have not yet entered the fusion site. Within a 15 minute time-frame, cells close to the zippering point further shorten their proximal junctions and become incorporated into the semi-rosette configuration around a common vertex (Figure 2). This process of junctional remodelling brings cells from the two sides of the neural folds into contact for the first time, converging at the shared site of integrin-mediated anchorage. Once in contact, cells within the semi-rosette establish new junctions with their contralateral counterparts, and then exit the semi-rosette into the region of surface ectoderm that overlies the closed neural tube. In this way, zippering is able to progress, as new cells bordering the open region undergo the same cycle of sequential constriction and semi-rosette formation.
Figure 2. Live imaging of surface ectoderm cells during zippering propagation. Individual cells shorten their proximal junctions over time (a) to form the semi-rosette configuration (c) and then exit the zippering point rostrally (d, e), with further cell elongation.
While the two classical models of epithelial fusion, contraction of an actomyosin cable and protrusion-mediated cell migration, are undoubtedly important in closure, the precise travel of the zipper over long distances, as in neural tube formation, cannot be explained by either of those mechanisms. Genetic or pharmacological disruption of the cytoskeleton does not halt spinal zippering (Escuin et al., 2015) and, while cellular protrusions make the first contacts at the zippering point (Rolo et al., 2016) we found they were intact in embryos lacking integrin b1. Zippering relies instead on small localised cell junctional rearrangements mediated by the action of integrin α5b1. Coordinated adhesion toward a common site of basal anchorage, probably involving fibronectin, brings pairs of contralaterally positioned cells into close proximity (Figure 3). This intermediate state of cell-ECM anchorage is crucial for the subsequent maturation and extension of novel cell-cell junctions between opposing cells, promoting closure and enabling progression of zippering.
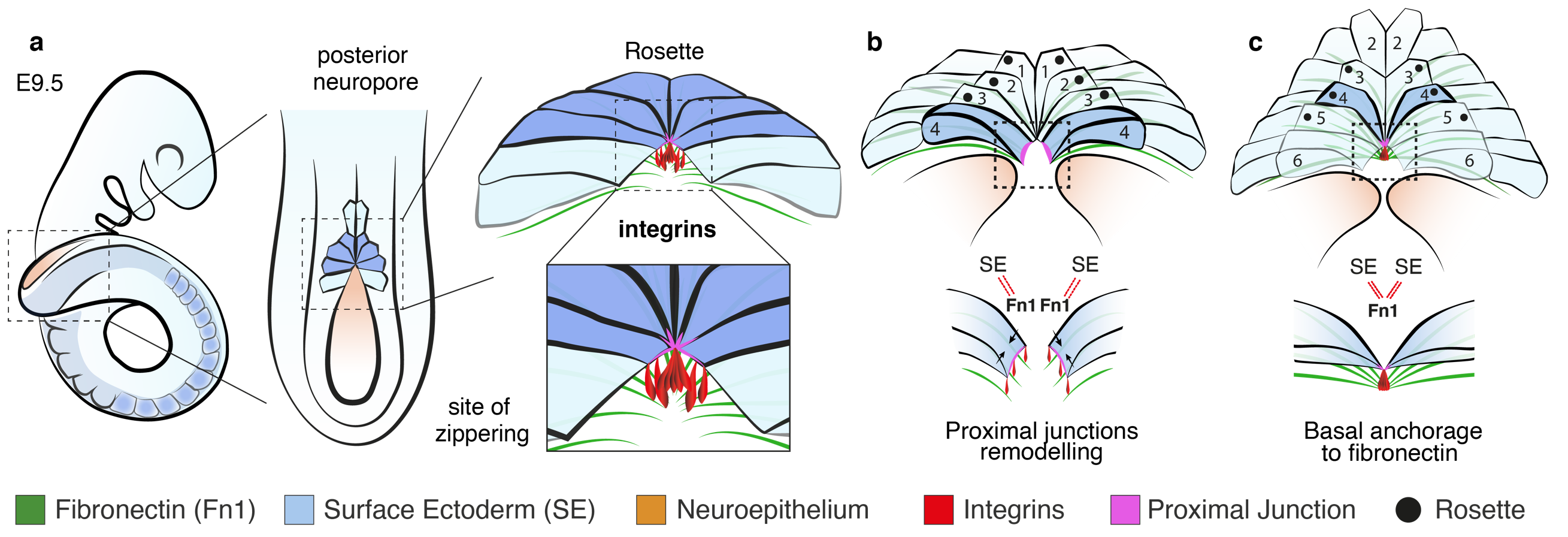
Indeed, a recent whole-exome sequencing study of families affected by NTDs identified variants in the integrin b1-encoding gene, ITGB1, among affected individuals, suggesting ITGB1 as a key predisposing gene in human NTDs (Lemay et al., 2018). The strong similarity between the open lesion in our mouse model and the condition of lumbo-sacral spina bifida in humans, emphasises the possibility that integrin-mediated anchorage may represent a conserved mechanism for neural tube zippering in humans as well as mice. Hence, impaired integrin function could represent a potentially significant risk factor in the aetiology of open spina bifida.
References:
Barczyk, M., Carracedo, S., and Gullberg, D. (2010). Integrins. Cell Tissue Res. 339, 269–280.
Greene, N.D.E., and Copp, A.J. (2014). Neural tube defects. Annu. Rev. Neurosci. 37, 221–242.