The right information
Posted by NietoLab, on 19 October 2017
Oscar H. Ocaña and M. Angela Nieto
Comment on “A right-handed signalling pathway drives heart looping in vertebrates”. Nature 549, 86-90 (2017).
A fundamental aspect of vertebrates is their external bilateral symmetry, which has to some extent shaped evolutionary success. Not only is beauty associated with symmetry, enhancing an individual’s chance of mating but also, symmetry in the legs will help an animal flee from a hunter (Enquist and Arak, 1994; Johnstone, 1994; Holló and Novak, 2012). However, a remarkable feature of the vertebrate body beyond this external symmetry is the asymmetric disposition and morphology of the internal organs relative to the left-right (L-R) axis. These asymmetries are essential for optimal organ packaging and function, yet it has long remained a mystery as to how this asymmetry is achieved. The initial symmetry is first broken in the vertebrate embryo at the level of the L/R organiser during early gastrulation. As a result, asymmetric information is transmitted to the lateral plate mesoderm (LPM), restricting the expression of Nodal and its downstream target Pitx2 to the left side of the embryo. The Nodal-Pitx2 pathway, which is conserved in all vertebrates, is instrumental in controlling L/R asymmetry (Raya and Izpisua Belmonte, 2006). This left-handed information is repressed on the right-hand side by the inducer of the epithelial-mesenchymal transition (EMT), Snail1. It has remained unclear whether an equivalent right-handed pathway provides instructive information to the right LPM. Laterality defects are associated with some important diseases in humans. Thus, the mechanisms underlying the establishment of L/R asymmetry are clearly of interest to developmental biologists and fully understanding these events will have significant biomedical implications.
New Findings
The mechanisms that regulate the EMT in development and disease have for long been of particular interest to our lab. When screening for genes expressed in the LPM of chick embryos, we found the Prrx1 transcription factor to be a particularly potent inducer of the EMT, and like Snail1, not only in embryos but also in cancer cells (Ocaña et al., 2012). When the expression of these genes was studied in more detail, we realised that Prrx1 is transiently expressed asymmetrically in the same temporal window as Snail1. Moreover, and as we also described previously for Snail1 (Morales et al., 2007), the expression of Prrx1 was stronger on the right than on the left side of the embryo. Likewise, prrx1a transiently appears to be asymmetrically distributed in zebrafish embryos during a similar developmental window as in the chick embryo, again with higher levels on the right-hand side. Therefore, with great expectation, we tested whether the asymmetric distribution of Prrx1 influenced organ laterality, focusing on heart positioning as this is the first clear indication of morphological L/R asymmetry in the embryo. The fastest and easiest way to tackle this question was to knock-down prrx1a expression in the zebrafish and we found that at 48 hpf, the majority of the morphant embryos developed mesocardia and the dextral looping typically observed in embryos was completely abrogated. To determine whether this effect was conserved in chicken, we performed loss of function experiments by electroporating RNAi against Prrx1 bilaterally into cardiomyocyte precursors. Prrx1 downregulation provoked a similar effect, with heart mesocardia being the main phenotype observed. Thus, it seemed that in both the fish and chicken asymmetric Prrx1 expression was required for heart laterality.
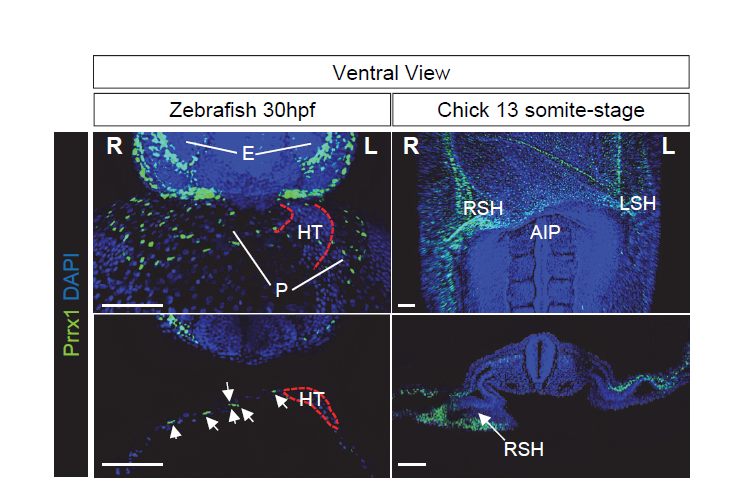
Given the conserved asymmetric expression of Prrx1, stronger on the right-hand side, and its role during heart looping, we reasoned that an instructive pathway may convey information to the right-hand side, in addition to the left pathway. Support for the existence of a right-handed program came from studies into mild Snail1 downregulation on the right-hand side, which provoked heart looping defects in the chick embryo without affecting Pitx2 expression (Patel et al., 1999). In addition, the development and position of the proepicardium, a transient specifically right-sided structure in frogs and avians, was affected by altered Snail1 expression but it was not after aberrant bilateral Pitx2 expression (Schlueter and Brand, 2009). Furthermore, zebrafish and mice carrying mutations in Pitx2 do not display heart looping defects (Campione et al., 2001; Ji et al., 2016). Together, these data suggest that heart laterality may be driven by a dominant right-handed pathway. As such, we speculated that reversing the levels of Prrx1 expression, so that higher levels were expressed on the left rather than the right-hand side, would lead to the development of embryos with reverse looping.
To test our hypothesis, we took advantage of the electroporation technique that allows unilateral manipulation of gene expression in chick. We studied the effect of Prrx1 gain-of-function in the left LPM and we found that a significant proportion of the embryos displayed reverse heart situs. These data indicate that the transiently stronger expression of Prrx1 in the right LPM was sufficient to drive heart looping. To gain further insight into the mechanism underlying this transient asymmetric expression of Prrx1 and how it affected heart looping, we started to precisely characterise the cell populations that contribute to heart development and that express this gene. This involved developing an antibody against Prrx1 and performing dual or multiple immunolabelling of cell populations associated with the heart using different markers, carrying out an exhaustive confocal analysis. As a result, we found that Prrx1 was not expressed in the primary heart tube (PHT) but that it was in fact expressed by a population of cells lateral and posterior to the cardiac venous pole.
The finding that another EMT inducer, in addition to Snail1, was strongly expressed in the right LPM, also influencing heart situs, suggestsed to us that some common features could exist between L-R pathways and the EMT program. As such, we were prompted to study whether differential L-R cell movements promoted by Prrx1 may drive heart looping. To investigate the movement and fate of the cells that express Prrx1 and that contribute to the posterior pole, new tools had to be generated and thus, we first addressed this question in the zebrafish. We generated a tbx5a-reporter transgenic line Tg(tbx5a:eGFP) as the cells expressing this gene contribute to both the PHT and the posterior pole of the heart (Ahn et al., 2002). In this tbx5a-reporter line there was complete co-localization between eGFP and Prrx1 in a subpopulation of the LPM cells, in the region of the cardiac precursors, which validated the use of these animals. When cell movements were followed in this reporter line, time lapse recordings of embryos from 28 to 48 hpf (during which time heart looping occurs) indicated an asymmetric migration of the Tbx5/Prrx1 double positive cells at the posterior pole of the heart. Significantly, there was a higher contribution of cells from the right than from the left-hand side, which correlates perfectly with the asymmetric expression of Prrx1 driving a different left/right cell contribution. In fact, prrx1a downregulation impaired this asymmetric cell migration of posterior pole cells and leads to mesocardia.
These observations raise the question as to whether these cells contribute to heart positioning and morphogenesis. Lineage tracing in zebrafish embryos demonstrated that Prrx1 expressing cells contribute to the PHT at the time of the heart looping, yet this Prrx1 expression is downregulated concomitant with their incorporation into the heart tube. Interestingly, the size of the atrium was reduced in the Prrx1a morphants, indicating that Prrx1 expression by cardiac progenitors is also required for heart morphogenesis.
The heart looping defects observed when Prrx1 was downregulated were compatible with studies describing the asymmetric contribution of cells from the right and left of the embryo to the heart after the formation of the PHT (Taber et al., 2010; Dominguez et al., 2012). Thus, we thought that differential left-right cell movements driven by Prrx1 could generate asymmetric forces and tension that would be stronger from the right. Such forces might eventually lead to the initial leftward bending of the posterior pole of the heart and the subsequent dextral torsion. In accordance with this hypothesis, cells in the tbx5a reporter could be seen migrating from the right-hand side to the posterior pole at the heart looping stage, apparently forming a structure reminiscent of a cable. Since forces in developing tissues are usually controlled by actomyosin bundles, we visualized actin stress fibres in vivo and while cardiac looping was normally accompanied by the formation of an actomyosin cable directed towards the posterior pole, this was not the case in the Prrx1a morphant embryos. Moreover, laser ablation of Prrx1/Tbx5 expressing cells on the right but not on the left side of the embryo prevented heart lateralization. These experiments demonstrate that asymmetric tension, more intense on the right-hand side, drives heart looping. Interestingly, we found that this mechanism was conserved in the chick embryo. Collectively, these data indicate that as in the fish, leftward displacement of the posterior pole and the subsequent dextral looping is driven by an actomyosin-dependent mechanism.
Having found that the asymmetric expression of Prrx1 plays a key role in heart lateralization, both in zebrafish and chick embryos, the next issue was to place this transcription factor it in the signalling pathways already known to be involved in L/R asymmetry. We knew that BMP could induce Prrx1 expression in the chicken embryo LPM (Ocaña et al., 2012), where it also induces Snail1 to repress Pitx2 expression (Raya and Izpisua Belmonte, 2006). In loss- and gain-of-function experiments performed on zebrafish and chick embryos, we confirmed that Prrx1 was activated by BMP and repressed by Nodal signalling. Hence, heart looping is driven by a BMP-mediated pathway that promotes strong Prrx1 expression, and that is repressed on the left by Nodal.
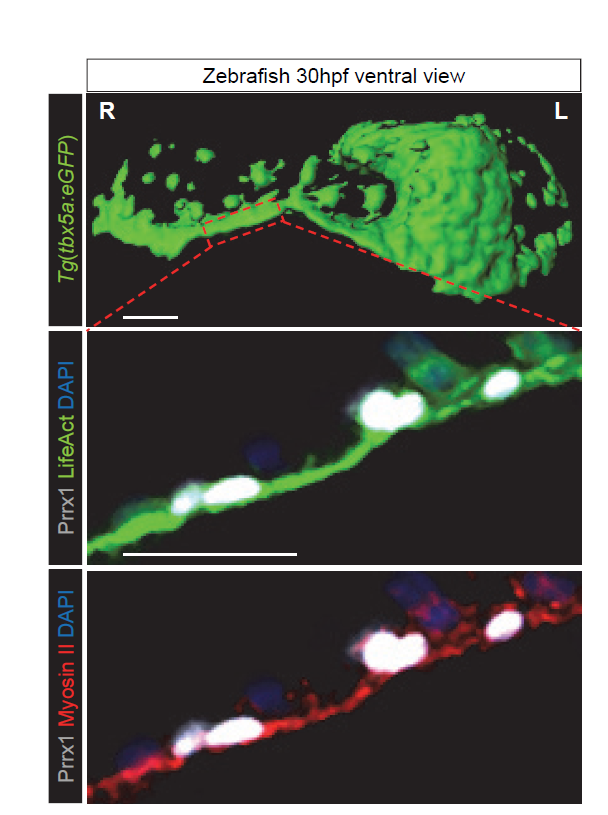
A surprising evo-devo twist
Having shown the implication of Prrx1 in heart looping in both the fish and chick embryo, we turned our attention to the mouse embryo. At first, it was disappointing that our model did not seem to fit well, as there was no heart looping defect evident in Prrx1 mutant mice (Bergwerff et al., 2000). However, we knew that Snail1 mutants develop heart looping defects (Murray and Gridley, 2006) and thus, we compared Prrx1 and Snail1 expression side-by-side in mouse and chick embryos. In the territories relevant to heart looping, we found an interchange of expression patterns between these two EMT inducers (Prrx1 and Snail1). While chick embryos displayed asymmetric Prrx1 expression in the ventral posterior pole of the heart, mice displayed asymmetric Snail1 expression along with an accumulation of F-actin fibres in this territory, but no Prrx1 expression. As such, these results offered a convincing explanation for the heart laterality defects observed in Snail1 mutant mice and the lack of such defects in the Prrx1 mutants. Thus, in terms of heart laterality, it is Snail1 in the mouse that seems to carry out the role of Prrx1 in the fish and chick. While this observation may appear surprising, we had previously observed other interchanges in the lab, such as those between Snail1 and Snail2 expression at other sites in the chick and mouse embryos (Locascio et al., 2002).
General conclusions
We have identified a prominent right-handed pathway that is driven by BMP signalling and that is in turn repressed on the left-hand side by Nodal. This right-hand signalling induces asymmetric L/R activation of different EMT transcription factors, Prrx1 in fish and chick, and Snail1 in the mouse embryo, which provoke asymmetric cell movements and forces that are stronger on the right-hand side of the embryo. In fact, these asymmetric forces induce the leftward displacement of the posterior pole and dextral looping of the heart in an actomyosin-dependent manner. We are very excited as to how these studies have progressed, as they have allowed us to unravel a basic mechanism that has been conserved in vertebrates and that controls heart looping. Furthermore, this mechanism could help us better understand the congenital heart diseases that are related to heart laterality in humans.
References
Ahn, D. G., et al. (2002), ‘T-box gene tbx5 is essential for formation of the pectoral limb bud’, Nature 417, 754–758.
Bergwerff, M., et al. (2000), ‘Loss of function of the Prx1 and Prx2 homeobox genes alters architecture of the great elastic arteries and ductus arteriosus’, Virchows Arch, 436 (1), 12-9.
Campione, M., et al. (2001), ‘Pitx2 expression defines a left cardiac lineage of cells: evidence for atrial and ventricular molecular isomerism in the iv/iv mice’, Dev Biol, 231 (1), 252-64.
Dominguez, J. N., et al. (2012), ‘Asymmetric fate of the posterior part of the second heart field results in unexpected left/right contributions to both poles of the heart’, Circ Res, 111 (10), 1323-35.
Enquist, M. and Arak, A. (1994), ‘Symmetry, beauty and evolution’, Nature, 372, 169-72.
Holló, G. and Novák, M. (2012), ‘The manoeuvrability hypothesis to explain the maintenance of bilateral symmetry in animal evolution’, Biol Direct, 7:22.
Ji, Y., Buel, S. M., and Amack, J. D. (2016), ‘Mutations in zebrafish pitx2 model congenital malformations in Axenfeld-Rieger syndrome but do not disrupt left-right placement of visceral organs’, Dev Biol, 416 (1), 69-81.
Johnstone, R.A. (1994), ‘Female preference for symmetrical males as a by-product of selection for mate recognition’, Nature, 372, 172-5.
Locascio, A., et al. (2002), ‘Modularity and reshuffling of Snail and Slug expression during vertebrate evolution’, Proc Natl Acad Sci U S A, 99 (26), 16841-6.
Morales, A. V., et al. (2007), ‘Snail genes at the crossroads of symmetric and asymmetric processes in the developing mesoderm’, EMBO Rep, 8 (1), 104-9.
Murray, S. A. and Gridley, T. (2006), ‘Snail family genes are required for left-right asymmetry determination, but not neural crest formation, in mice’, Proc Natl Acad Sci U S A, 103 (27), 10300-4.
Ocaña, O. H., et al. (2012), ‘Metastatic colonization requires the repression of the epithelial-mesenchymal transition inducer Prrx1’, Cancer Cell, 22 (6), 709-24.
Patel, K., Isaac, A., and Cooke, J. (1999), ‘Nodal signalling and the roles of the transcription factors SnR and Pitx2 in vertebrate left-right asymmetry’, Curr Biol, 9 (11), 609-12.
Raya, A. and Izpisua Belmonte, J. C. (2006), ‘Left-right asymmetry in the vertebrate embryo: from early information to higher-level integration’, Nat Rev Genet, 7 (4), 283-93
Schlueter, J. and Brand, T. (2009), ‘A right-sided pathway involving FGF8/Snai1 controls asymmetric development of the proepicardium in the chick embryo’, Proc Natl Acad Sci U S A, 106 (18), 7485-90.
Taber, L. A., Voronov, D. A. and Ramasubramanian, A. (2010), `The role of mechanical forces in the torsional component of cardiac looping´. Ann. N.Y. Acad. Sci. 1188, 103-110.