The people behind the papers – Joe Shawky & Lance Davidson
Posted by the Node Interviews, on 24 October 2018
The construction of complex three-dimensional tissue structures during embryogenesis requires precise control of cell and tissue mechanics. The Xenopus embryo provides a powerful tool for interrogating this relationship, as demonstrated by a recent Development paper reporting the use of tissue explants to test predictions of mechanical models. We caught up with first author and recent graduate Joseph Shawky and his supervisor Lance Davidson, Professor in the Department of Bioengineering in the University of Pittsburgh, to hear more about the work.
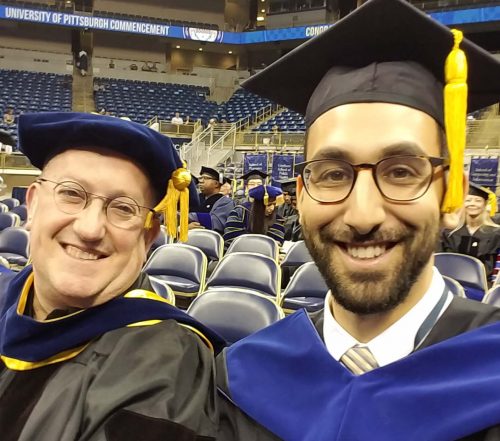
Lance, can you give us your scientific biography and the questions your lab is trying to answer?
LD I arrived as a stranger in a strange land. My interests as a kid were a mix of collecting (perfect for biology) and astronomy (perfect for making). As many of my generation I wanted to be an astronaut and focused on physics and math through a BS in Physics at the University of Illinois and an MS in Space Science from York University in Toronto. I exited the academic stream for several years working as a scientific analyst in the nascent field of data science, but returned to earn a PhD in Biophysics from UC Berkeley. While at Berkeley I encountered radicals looking at the many roles of mechanics in biology, from the physics of virus capsid formation to the impact of waves on coastal kelp forests to the mechanics of sea urchin gastrulation. I had arrived in Berkeley as a theorist and left as an experimentalist. In my postdoc I cross-trained in amphibian embryology and cell biology. My mentors, Ray Keller and Doug DeSimone, were incredibly generous with training and tolerant of my digressions. They let me roam the embryo working on various processes ranging from neurulation to wound healing. In 2006 after an extended 8 year trip through the embryo, I took a faculty position in Bioengineering at the University of Pittsburgh. My group sits squarely between several cell and developmental biology labs and have we have terrific colleagues both at the University and at our neighbour institutions, Carnegie Mellon University.
We have two main questions in the lab and one area for technology development. The first question is “how do embryos work?” and by that I really mean the physical principle of work (force times distance), how forces are generated and how they shape tissues. My early experience as a theorist left me unsatisfied with the state of experimental embryology and we have consistently worked to develop tools and conceptual working models of morphogenesis. The second question is “why is development so robust?” This is a newer question to our group where we seek to understand how regulative programs of development integrate mechanical cues with conventional signalling and gene expression networks. This is an exciting new area for us and is leading in some surprising directions. The last topic, is much more focused on turning principles of development into tangible tissue engineering tools. The remarkable events of morphogenesis continually put tissue engineers to shame and we are trying to bridge that gap, both by training tissue engineers in concepts from morphogenesis, and in developing microfabrication tools to mimic embryonic tissue self-assembly.
And Joe, how did you come to join the Davidson lab, and what drives your research?
JS I joined the Davidson lab as an eager graduate student fascinated by the world of cell and tissue mechanics. It was through the research opportunities I had during my undergraduate and master’s studies that I became interested in pursuing my PhD in the field of developmental biomechanics. I was first introduced to cell culture during my undergraduate studies at Rensselaer Polytechnic Institute (RPI) where I investigated potential therapies for breast cancer. From there, I continued to study breast cancer at Cornell University but in a different context. I studied how cancer cells reorganize their extracellular matrix during cell migration. Here, I was introduced to techniques to measure forces and stresses on the nanometer scale. I became very interested in the various approaches to study cell mechanics and the ability to link mechanical properties with biological phenomena. When I was deciding where to complete my graduate work, I was impressed by the various devices and techniques developed in house at the Davidson Lab to probe cell and tissue mechanics and was also excited to study tissue mechanics within developing embryonic tissues.
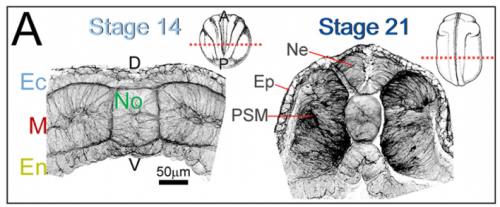
Can you introduce the basics of the cellular solids model? Why are such material models useful for studying embryos?
JS&LD The cellular solids model (CSM) is a theoretical framework that relates the stiffness of cellular materials to the microstructure of component cells. CSM was developed to characterize the mechanical properties of open- and closed-cell materials, both synthetic and biological in origin. CSM describes how factors such as cellular density, cell wall thickness and mechanical material properties affect the bulk stiffness of cellular materials. In our study, we tested the basic premise of the CSM to investigate how different features including cell size and cortex properties might affect the bulk mechanical properties of embryonic tissues. This model was useful because it makes predictions and allowed us to test the contribution of features at different size scales to critical bulk properties. However, at its finest scale the basic equations of the CSM do tell us how F-actin microstructure, including organization and degree of cross-linking, may be influencing mechanical material properties. Such mechanical models are key to understanding the direct role of mechanics in shaping tissues as well as the indirect role of mechanical cues in providing positional information for development.
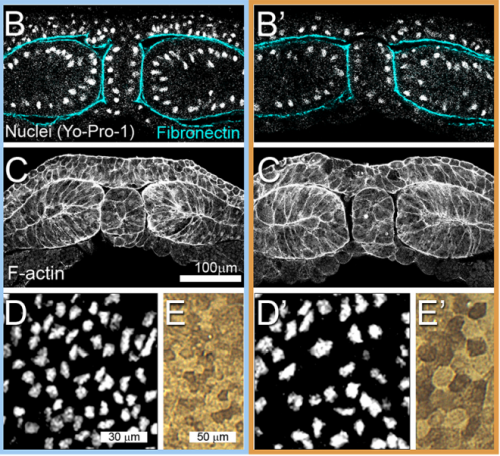
Can you give us the key results of the paper in a paragraph?
JS&LD In this study, our goal was to better understand the factors that contribute to bulk mechanics in Xenopus dorsal isolates and to shed light on possible mechanisms of stiffening during development. To formally make predications, we adapted the cellular solids model to embryonic tissues to predict how factors at different size scales may influence mechanics. We first confirmed the central assumption of the CSM, that tissue modulus does not depend on embedded structural elements, by engineering and mechanically testing a tissue devoid of large coherent 3D structures. We found that large-scale tissue architecture and cell size are not likely to influence bulk mechanical properties of early embryonic tissues. Our findings suggest that regulation of F-actin cortical thickness, density, and integrity plays a central role in regulating the physical mechanics of embryonic multicellular tissues.
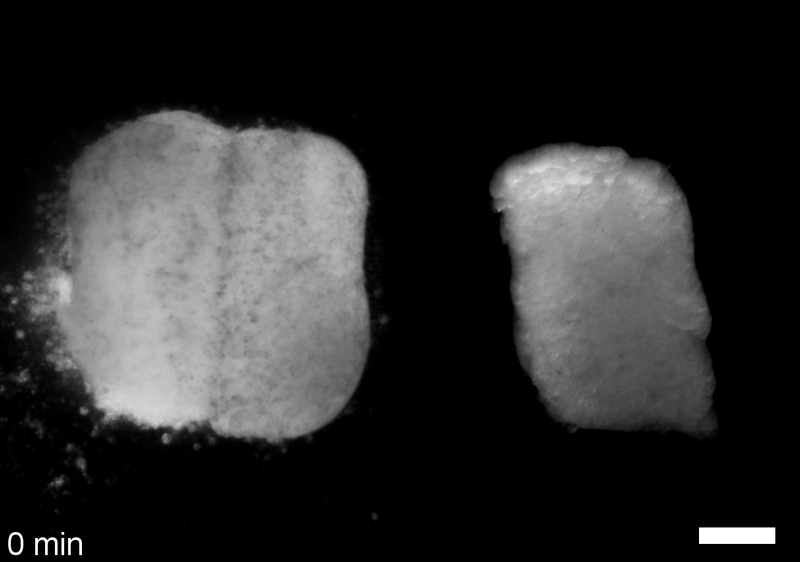
What do you think explains the failure of ‘scrambled’ tissue explants to stiffen with age when cultured?
JS&LD Scrambled tissues were not able to reorganize or stiffen to the full extent of native tissues over time, suggesting that the organization of germ layers or germ layer tissue shape might be important for mechanical maturation. It remains unclear what aspects of architecture facilitate stiffening however could be organization of the ECM or in response to bulk morphogenesis movements. Fibronectin, the most abundant ECM protein during gastrulation and neurulation, does not directly contribute to mechanical properties as shown in a previous study from our lab however ECM may serve to align cells within the native stress field and lead to mechanical maturation.
Your paper seems exemplary of a very exciting time for mechanics in developmental biology. What are the main unanswered questions we should be tackling in the next decade?
(1) We have sparse experimental understanding of the mechanics of morphogenesis, gleaned from only a few model systems (fly, worm, frog, chick) with mechanically-accessible tissues (e.g. Drosophila epithelium, Xenopus explants), but know little about the lesions in human development that drive structural birth defects (e.g. spina bifida, congenital heart defects, cleft palate). How do these defects arise and are their ways they can be predicted and prevented?
(2) The mechanical microenvironment within embryos is largely hidden and has been the subject of long speculation but seldom the subject of rigorous experimental approaches. New technologies are removing the veil from these properties and allow us to start describing mechanical cues in embryos and testing how they may be involved in patterning.
(3) The field of tissue engineering arose from the needs of surgeons to reconstruct organs and tissues when regeneration and endogenous programs of healing fall short. More recently tissue engineers have begun to realize the remarkable robustness of developing tissues and organs. Harnessing evolved programs of self-assembly would open up an entirely new field where tissues and biological structures could be designed from the ground up in the same manner that electrical engineers design new circuits and civil engineers design new bridges. Co-opting the ‘technology’ of development would transform basic research, human health, and establish whole new industries.
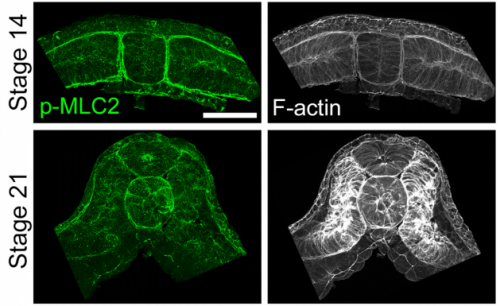
When doing the research, did you have any particular result or eureka moment that has stuck with you?
JS One of the exciting moments in the lab was when we developed a process to generate viable and reproducible scrambled tissues. It took several design iterations and hours in the lab’s machine shop to develop a chamber and protocol that worked. These experiments were particularly exciting because it was an out of the box approach that allowed us to flip our research question on its head and tackle the question using a novel technique.
And what about the flipside: any moments of frustration or despair?
JS Early on it was challenging to identify techniques to alter cell size, particularly decreasing cell size, without disrupting development. We tried several approaches including removing cytoplasm from the single cell embryo. Once we got focused on manipulating cell cycle we were able to find reliable ways to inhibit and accelerate the cell cycle and generate tissues with increased and decreased cell density.
So what next for you Joseph after this paper?
JS I’ve since graduated from the Davidson lab and completed a medical device policy fellowship at the U.S. Food and Drug Administration. Now, I am currently working as a Strategy and Analytics Consultant supporting clients within government health agencies.
Where will this work take the Davidson lab?
LD The Xenopus embryo provides unparalleled access to the mechanics of morphogenesis and allows us to test ideas that folks working with other models would think preposterous. By focusing on the most elementary of tissue movements, convergent extension of the dorsal axis, we have been free from confounding changes in cell identity and 3D reorganizations that drive more complex morphogenesis movements. We are now applying tools and theoretical frameworks developed from this work to expose cryptic mechanical cues and their roles in shaping organs such as the heart, skin, and cardiovascular network.
Finally, let’s move outside the lab – what do you like to do in your spare time in Pittsburgh?
LD I enjoy early morning dog walks through the forested city parks of Pittsburgh and lead a domestic life of cooking and gardening, but really I love spending free time in the lab since it is full of microscopes, mechanical gadgets, and frogs and there are so many open questions about embryonic development.
JS My favorite things to do in Pittsburgh are long bike rides on the beautiful riverside trails and enjoying the vibrant restaurant scene. Aside from biking and eating, I also enjoy grilling, hanging out with friends and a good podcast.
Multiscale analysis of architecture, cell size and the cell cortex reveals cortical F-actin density and composition are major contributors to mechanical properties during convergent extension. Joseph H. Shawky, Uma L. Balakrishnan, Carsten Stuckenholz, Lance A. Davidson
Development 2018 145: dev161281 doi: 10.1242/dev.161281
This is #51 in our interview series. Browse the archive here.