Sarah E. Hall
Department of Biology, Syracuse University, Syracuse, NY 13244
For over a century, the nature versus nurture debate has questioned the relative contributions of genetic sequences and the environment to the phenotype of an individual (Galton 1869). Genome-wide association studies in humans have shown that environmental stress experienced in utero or during critical periods of development can affect the physiology of adults, even in the absence of the original stress in future generations (e.g. Kaati et al. 2007). The mechanism of this phenomenon, known as environmental programming, is through stress-specific modulation of the hippocampal-pituitary-adrenal (HPA) axis by epigenetic regulation of genes important for the mammalian stress response (Spencer 2013). Although some progress has been made in the mechanistic understanding of environmental programming over the last decade, some unanswered questions still remain. How does environmental stress globally influence the epigenome to affect development and behavior in animals? Do these environmentally programed changes in gene expression have evolutionary consequences?
To address these questions, we developed Caenorhabditis elegans as a model system of environmental programming as their developmental trajectory is dependent upon their environmental conditions in early life. If conditions are favorable for growth after hatching (available food, low population density), worms will develop continuously through four larval stages to become a reproductive adult (controls). However, if food is scarce or population density is high (detected by high levels of pheromone), worms can enter the dauer diapause stage (Cassada and Russell 1975). When dauers detect more favorable environmental conditions during migration, they can re-enter continuous development to become reproductive postdauer adults. Since C. elegans populations are primarily self-fertilizing hermaphrodites producing isogenic progeny, they offer the opportunity to directly examine the environmental regulation of gene expression.
Our initial experiments examining differences between wildtype control and postdauer adults that experienced crowding showed significant effects on gene expression profiles, genome-wide chromatin states, and life history traits such as longevity and reproduction (Hall et al. 2010). In addition, we found that endogenous small interfering RNA (endo-siRNA) sequences differed between the two populations, and that the argonaute CSR-1 was a major contributor to the environmental programming of postdauer phenotypes (Hall et al. 2013). After starting my own laboratory in 2012, we began follow-up experiments of my initial results, particularly brood size assays, to dissect the mechanism of environmental programming in C. elegans. To my surprise, we were getting the opposite results from what was expected—a feeling that a starting assistant professor does not want to experience! At the same time, a graduate student in my lab was examining the role of endogenous RNAi pathways in dauer formation, and was finding that mutations in RNAi components exhibited stress-specific effects on dauer formation and were required in different tissue types (Bharadwaj and Hall 2017). Together, these results led us to the realization that perhaps not all dauers are created equally, and that different early-life stresses may lead to distinct adult phenotypes. Our most recent study, “Early experiences mediate distinct adult gene expression and reproductive programs in Caenorhabditis elegans” published in PLoS Genetics, was born out of this new hypothesis.
To examine if different dauer-inducing stresses result in distinct adult phenotypes, analogous to what is observed in humans, we performed transcriptional profiling of control and postdauer adults that experienced either starvation or crowding (pheromone)-induced dauer in wild type and csr-1 hypomorph strains (Ow et al. 2018). In both wildtype datasets, we found over 1000 genes that exhibited significant changes in gene expression between postdauer and control adults. To our surprise, we found few genes whose expression were affected by dauer formation generally. Instead, we identified a set of 512 genes whose gene expression was opposite in postdauers compared to controls depending on the dauer-inducing stress (Figure 1). After careful validation using multiple biologically independent samples to verify that we did not just mix up the tubes, we were thoroughly convinced and named this set of genes the “seesaw” genes based on their up-or-down, stress-sensitive changes in gene expression. Curation of these genes showed that their expression patterns correlated with their stress-specific changes in mRNA levels between controls and postdauers. For example, in the starvation condition, genes expressed in somatic tissue were upregulated while genes expressed in the germ line were downregulated, and vice versa for crowding. The magnitude and pattern of these gene expression changes are so striking that it would be possible to determine the life history of worm just by measuring the mRNA levels of a few genes!
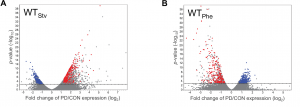
Figure 1 Early life history experience mediates gene expression in adults. Volcano plots representing mRNA changes between wild-type control and postdauer adults that experienced early-life (A) starvation or (B) crowding (pheromone) conditions. The dotted line indicates FDR cutoff of p = 0.05. Red and blue dots represent the WTPhe down::WTStv up and WTPhe up::WTStv down seesaw genes, respectively. This figure was originally published as Figure 1 A and B in Ow et al. (2018).
To examine how endogenous RNAi pathways may be playing a role in environmental programming, we also examined the transcriptional profiles of csr-1 hypomorph control and postdauer adults that experienced different stresses. Interestingly, we found that seesaw changes in gene expression were almost entirely eliminated in the csr-1 hypomorph, including for somatic genes that have not yet been identified as CSR-1 targets (Figure 2). The argonaute CSR-1 has been implicated in numerous mechanisms regulating gene expression, including promoting transcription and formation of euchromatic domains, post-transcriptional gene silencing, and inhibition of translation (Wedeles et al. 2013). Most of our knowledge of CSR-1 function is from studies within the germ line, including CSR-1’s germline expressed targets (Claycomb et al. 2009). However, these results suggest that CSR-1 may have more far-reaching effects on gene expression in tissues other than the germ line.
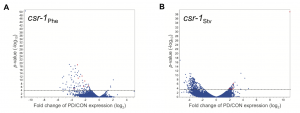
Figure 2 Environmentally programmed gene expression changes are dependent on CSR-1. Volcano plots depicting the mRNA changes between csr-1 hypomorph control and postdauer adults that experienced early-life (A) crowding or (B) starvation conditions. Dotted line indicates FDR cutoff p = 0.05. Red dots represent the csr-1Phe down::csr-1Stv up seesaw genes. This figure was originally published at Figure 2 in Ow et al. (2018).
Given the significant genome-wide effect we observed in the csr-1 hypomorph compared to wild type, we were curious whether CSR-1 was regulating the observed changes in mRNA at the transcriptional level. Since CSR-1 targets in the germ line have been shown to be physically clustered in the genome (Tu et al. 2015), we examined if there was a relationship between seesaw gene expression change and their chromosomal location. When we plotted the gene expression changes between control and postdauer adults for all genes in starvation or crowding conditions, we observed a striking trend indicating that a majority of genes exhibit seesaw expression, although not in the strict statistical sense of our transcriptional profiling analysis. When we mapped the seesaw genes to a chromosomal location, we were surprised to find that genes with similar changes in gene expression in the starvation conditions were found on the same chromosomes. For instance, genes that are upregulated in starvation-induced postdauers were enriched on chromosomes V and X, whereas genes that were downregulated in the same conditions were primarily enriched on chromosomes I and III (Figure 3). Since most isolated wild populations of C. elegans have been found in dauer (Frezal and Felix 2015), this observation suggests that gene expression changes in starvation-induced dauers are ecologically relevant, and perhaps beneficial, to the fitness of postdauers and may have contributed to the evolution of the C. elegans genome. This observation also is consistent with the model that CSR-1 could be regulating genes at the transcriptional level based on environmental cues. Since CSR-1 is thought to organize chromatin domains, selection may have favored clustering of genes with similar regulation in response to starvation so that chromatin remodeling of that “domain” could be more efficient. We are currently testing this hypothesis.
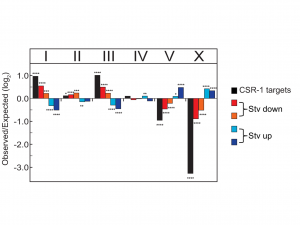
Figure 3 Chromosomal bias of CSR-1 targets and expression changes for all genes. Genes are categorized by quadrant analysis as shown in Figure 5A in Ow et al. (2018). * p < 0.05; ** p < 0.001; *** p < 0.001; **** p < 0.0001; χ2comparison to uniform distribution based on gene number per chromosome. This figure was originally published as Figure 5C in Ow et al. (2018).
To examine if the seesaw gene expression changes had consequences on fitness, we examined the evolutionarily selectable trait of brood size. We had previously shown that crowding-induced postdauers had a significantly larger brood size compared to control adults (Hall et al. 2010). Interestingly, the brood size seesaws similarly to gene expression, as starvation-induced postdauers have a significantly lower brood size compared to controls (Figure 4). Since C. elegans development is famously invariant (Kipreos 2005), we wondered about the developmental mechanism regulating the stress-specific changes in reproductive plasticity. Worm germ line development begins with mitotic cell proliferation maintained by the distal tip cell in early larval stages. As the gonad grows and expands, the cells pushed beyond the distal tip cell undergo meiosis, first becoming sperm during the L4 larval stage and then oocytes during adulthood (Kimble and White 1981; Austin and Kimble 1987). Given that self-fertilization in hermaphrodites is limited by the number of sperm produced, we hypothesized that changes in sperm number could result in altered brood size in adults.
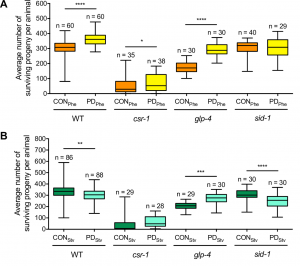
Figure 4 Reproductive plasticity is dependent on early life history. Whisker-box plots (minimum and maximum of all data) of the average brood size for controls (CON) and postdauers (PD) that experienced (A) high pheromone or (B) starvation for WT, csr-1 hypomorph, glp-4(bn2), and sid-1(qt9) strains. Assays for WT, the csr-1 hypomorph, and sid-1(qt9) were performed at 20ºC; brood assays for glp-4(bn2) were performed at 15ºC. Total sample number is indicated by n over at least 3 biologically independent trials. This figure was originally published as Figure 6A and B in Ow et al. (2018).
A major difficulty in developing this manuscript was how to test our brood size hypothesis. Our initial attempts to count mature sperm in young adult animals were unsuccessful due to high variability in sperm counts relative to the brood size differences between control and postdauer adults. Eventually, we decided to examine for differences in the onset of germline proliferation in early larval stages, with the expected result being that starvation-induced postdauers would initiate germline proliferation later, and crowding-induced postdauers earlier, compared to controls. Germline proliferation begins in the L3 larval stage. However, dauer animals bypass the L3 stage; thus, our challenge was to determine a method to synchronize the somatic development of control and postdauer larvae to compare the onset of proliferation. Since the vulva has distinct morphology characteristic of each developmental stage (Seydoux et al. 1993; Mok et al. 2015), we used vulva development as our method of synchronizing populations. Using DAPI-staining of whole-mount larvae, we were able to determine that control and postdauer worms at the identical stage of somatic development exhibited different numbers of germ cell rows. Our results indicated that crowding-induced and starvation-induced postdauer larvae exhibited significantly greater or fewer germ line cell rows, respectively, compared to controls. This observation was consistent with our hypothesis and the brood size differences in adult populations. From a developmental perspective, these results are logical given that larvae induced into dauer by starvation would have less fat stored compared to crowding-induced dauers. Dauer formation and exit is likely to be metabolically expensive, especially since dauers are non-feeding; thus, it may require more time for starvation-induced postdauers to acquire enough fat to begin reproduction. In addition, environmental stresses such crowding and starvation have been shown to negatively regulate germline proliferation in L4 control animals through modulation of Notch genes via TGF-ß signaling (Pekar et al. 2017; Dalfó et al. 2012). TGF-ß signaling also regulates dauer formation based on environmental cues (Fielenbach and Antebi 2008), and a connection between this pathway and the regulation of seesaw genes and the brood size phenotype remains to be determined. Why crowding-induced dauer formation results in an earlier onset of germline proliferation, and not a delay similar to starvation, is not clear. However, we can speculate that postdauer worms that were crowded, but not starved, may begin germline proliferation earlier to produce a few offspring before the food is depleted.
Although many questions still remain regarding the mechanisms regulating seesaw gene expression, we were able to determine some mechanistic points of our model by performing qRT-PCR and brood size analysis in control and postdauer adults of different mutant strains. First, our csr-1 hypomorph transcriptional profiling results evoked two non-mutually exclusive hypotheses to explain CSR-1 function in environmental programming: 1) CSR-1 dependent signals are exported from the germ line to regulate somatic gene expression, or 2) CSR-1 targets a unique set of genes in somatic tissues compared to the germ line. Using a glp-4(bn2) strain, which lacks a germ line at restrictive temperature, we determined that a functional germ line was only required for starvation-induced phenotypes, not crowding phenotypes, regardless of where the genes are expressed. This conclusion was also consistent with the brood sizes assays of glp-4 animals at the permissive temperature (Figure 4). Given that the seesaw changes appear to involve crosstalk between tissue types, and CSR-1 is in the endogenous RNAi pathway, we also examined whether systemic RNAi might play a role. Systemic RNAi involves the spread of double stranded RNAs between cell and tissue types, which ultimately results in the downregulation of the homologous mRNA (Winston et al. 2002; Feinberg and Hunter 2003). To our surprise, only the crowding-induced phenotypes required the effector of systemic RNAi, SID-1, suggesting the possibility that endogenous dsRNA may transmit signals across tissue types about environmental conditions (Figure 4). Thus, we derived a model whereby the starvation-induced phenotypes require signals from the germ line that are not dsRNA, and the crowding-induced phenotypes require systemic RNAi but not the germ line (Figure 5). This model indicates that CSR-1 can function in somatic tissue independently from the germ line for the crowding phenotypes. However, in the starvation condition, whether the germ line signal requires CSR-1 function remains unclear. Moreover, where the systemic RNAi and germline-dependent signals are being produced, where they are being received, and what genes they might be regulating to elicit the stress-specific phenotypes are yet to be determined.
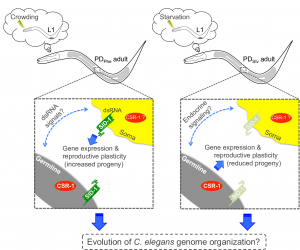
Figure 5 Postdauer animals retain a cellular memory of early environmental history that governs gene expression and reproductive plasticity. The germ line, SID-1, and CSR-1 mediate the crowding- and starvation-induced programs. This figure was originally published as Figure 7 in Ow et al. (2018).
An interesting aspect of this model is that the separate mechanisms for starvation and crowding induced phenotypes are in opposition, such that if you mutate one pathway (i.e. glp-4 for starvation phenotypes), the postdauer animals exhibit the phenotypes of the opposite stress (Figure 4). This observation suggests that starvation and crowding-induced mechanisms regulating the seesaw genes may share common components, but whether that pathway is in “starvation mode” or “crowding mode” is determined by stress-specific cues. Identification and dissection of these pathways will be critical in understanding how different stresses in early development can result in distinct adult phenotypes. Different early-life stresses in humans also result in different gene expression and phenotypic outcomes in adults; thus, we are more like worms than ever before.
Austin, Judithe, and Judith Kimble. “glp-1 is required in the germ line for regulation of the decision between mitosis and meiosis in C. elegans.” Cell 51.4 (1987): 589-599.
Bharadwaj, Pallavi S., and Sarah E. Hall. “Endogenous RNAi pathways are required in neurons for dauer formation in Caenorhabditis elegans.” Genetics 205.4 (2017): 1503-1516.
Cassada, Randall C., and Richard L. Russell. “The dauerlarva, a post-embryonic developmental variant of the nematode Caenorhabditis elegans.” Developmental biology 46.2 (1975): 326-342.
Claycomb, Julie M., et al. “The Argonaute CSR-1 and its 22G-RNA cofactors are required for holocentric chromosome segregation.” Cell 139.1 (2009): 123-134.
Dalfó, Diana, David Michaelson, and E. Jane Albert Hubbard. “Sensory regulation of the C. elegans germline through TGF-β-dependent signaling in the niche.” Current Biology 22.8 (2012): 712-719.
Fielenbach, Nicole, and Adam Antebi. “C. elegans dauer formation and the molecular basis of plasticity.” Genes & development 22.16 (2008): 2149-2165.
Feinberg, Evan H., and Craig P. Hunter. “Transport of dsRNA into cells by the transmembrane protein SID-1.” Science301.5639 (2003): 1545-1547.
Frézal, Lise, and Marie-Anne Félix. “The natural history of model organisms: C. elegans outside the Petri dish.” Elife 4 (2015): e05849.
Galton, Francis. Hereditary genius: An inquiry into its laws and consequences. Vol. 27. Macmillan, 1869.
Hall, Sarah E., et al. “A cellular memory of developmental history generates phenotypic diversity in C. elegans.” Current Biology 20.2 (2010): 149-155.
Hall, Sarah E., et al. “RNAi pathways contribute to developmental history-dependent phenotypic plasticity in C. elegans.” Rna 19.3 (2013): 306-319.
Kaati, Gunnar, et al. “Transgenerational response to nutrition, early life circumstances and longevity.” European Journal of Human Genetics 15.7 (2007): 784.
Kimble, J. E., and J. G. White. “On the control of germ cell development in Caenorhabditis elegans.” Developmental biology 81.2 (1981): 208-219.
Kipreos, Edward T. “Developmental cell biology: C. elegans cell cycles: invariance and stem cell divisions.” Nature Reviews Molecular Cell Biology 6.10 (2005): 766.
Mok, Darren ZL, Paul W. Sternberg, and Takao Inoue. “Morphologically defined sub-stages of C. elegans vulval development in the fourth larval stage.” BMC developmental biology 15.1 (2015): 26.
Ow, Maria C., et al. “Early experiences mediate distinct adult gene expression and reproductive programs in Caenorhabditis elegans.” PLoS genetics 14.2 (2018): e1007219.
Pekar, Olga, et al. “Linking the environment, DAF-7/TGFβ signaling and LAG-2/DSL ligand expression in the germline stem cell niche.” Development 144.16 (2017): 2896-2906.
Seydoux, Geraldine, Cathy Salvage, and Iva Greenwald. “Isolation and characterization of mutations causing abnormal eversion of the vulva in Caenorhabditis elegans.” Developmental biology 157.2 (1993): 423-436.
Spencer, Sarah J. “Perinatal programming of neuroendocrine mechanisms connecting feeding behavior and stress.” Frontiers in neuroscience 7 (2013): 109.
Tu, Shikui, et al. “Comparative functional characterization of the CSR-1 22G-RNA pathway in Caenorhabditis nematodes.” Nucleic acids research 43.1 (2014): 208-224.
Wedeles, Christopher J., Monica Z. Wu, and Julie M. Claycomb. “A multitasking Argonaute: exploring the many facets of C. elegans CSR-1.” Chromosome research 21.6-7 (2013): 573-586.
Winston, William M., Christina Molodowitch, and Craig P. Hunter. “Systemic RNAi in C. elegans requires the putative transmembrane protein SID-1.” Science 295.5564 (2002): 2456-2459.
(1 votes)
Loading...