The winner Daniyal Jafree is a medical 1st year PhD student working on the project “Unravelling the origins of the kidney lymphatics” in the group of Dr David Long at UCL. In his piece he writes about a paper by Paul Riley from 2015 which addresses the development and function of the cardiac lymphatic system. Danyial’s piece is a wonderful example of how good DB research has induced a paradigm shift in the cardiac field, but also profoundly changed the career of a young researcher. As Danyial writes at the end: “This paper inspired me so much that I contacted Professor Riley to ask whether he had any free positions in this lab. Sadly, he didn’t. But, funnily enough, I am now tackling a PhD in lymphatic biology at my own university, integrated into my medical degree. And guess who I’m collaborating with!”
Laura Hankins (runner up; Dunn school, Oxford) relates childhood memories of observing newts at the pond with the transplantation experiments performed in newts by Hilde Mangold and Hans Spemann – the experiments that sparked Laura’s interest in Dev Biol. She reminds us of the fact that our science is more than the focus on disease and sustainability, but concerns true biology and the wonders of nature around us. And she alerts our technology-focussed minds to the fact that there is an art and beauty in experimental design whatever method we use. As Laura comments towards the end: “This experiment is inspiring partly due to the minimalism of its approach; it demonstrates that the most influential experiments are designed without unnecessary embellishment.”
Victoria Rook (runner up; PhD at Queen Mary, London) takes a very different, critical view at the future, elegantly framed by comparing current developments in cloning and the use of chimerae and genomic engineering to the dystopian science fiction book “Oryx and Crake” by Margaret Atwood. Weighing optimism against pessimism, she ends with the words: “Soon we will have the resources to cure numerous genetic diseases and, in theory, the ability to improve the lives and health of generations to come. The unease comes with how far we are liable to take this, is a dystopian future where ‘pigoons’ and ‘crakers’ run wild within our reach, or will they remain a thing of fiction?”
See also a selection further submissions:
Kane Toh Qin uses Conrad Waddington epigenetic landscape proposed in the 1940s as an example to project from the past to the presence and beyond.
Emilio Mendez describes how learning about limb bud transplantation experiments performed by Ross G. Harrison in the 1920 inspired his passion for Developmental Biology.
Amanda Berg looks at the future of humans in space and the colonisation of other planets, and the need to investigate the possibility of reproduction and embryonic development away from earth.
Caitlin McQueen describes how she was influenced by the publications on nuclear transfer experiments carried out by John Gurdon in Xenopus laevis intestinal cells.
Massimo Ganassi talks about the difficulty and importance of communicating our science.
Anna Klucnika alerts to the need of communicating DB and provides some thoughts how to do it.
Sandra G González Malagon asks the fundamental question of what DB has contributed to society.
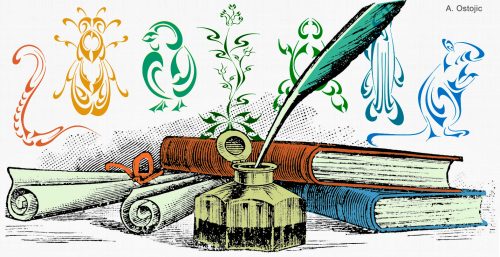
Essay question: The experiment/paper in Developmental Biology that most inspired you (winner)
Daniyal Jafree
As a medical student into Developmental Biology, it bugged me when my friends asked: “Why Developmental Biology? That’s boring, and not relevant to medicine at all?” For me, there is no paper that disproves this greater than that published by Paul Riley and colleagues from the University of Oxford, in Nature in June 20151. This paper, representing eight years of work, examined the development and function of the cardiac lymphatics. Together with two other papers published at around the same period, Riley and his group overturned a 100-year old dogma in lymphatic biology within the space of about 12 months, and laid the foundation for a new therapeutic strategy for heart disease.
Lymphatic biology is a very hot topic. These vessels, at the interface between vascular and immune systems, have been implicated in cancer, obesity, hypertension, inflammatory diseases and beyond. Lymphatics supposedly arise from a single source. A subset of cells in the wall of the cardinal vein express markers of lymphatic fate specification early in development. These cells bud off, form lymphatic sacs, and reach out to produce the entire lymphatic system. At least that is what we thought.
Riley and his group performed Cre-based lineage tracing to capture the venous-derived lymphatics in the heart. Remarkably, not all of the cardiac lymphatics were labelled. So where on earth were these non-venous derived cells coming from? The group went ‘all out’, using a battery of Cre lines in a painstaking effort to capture these mysterious cells. The answer was shocking: they were not coming from the heart, nor the embryo at all! They were coming from outside the embryo, from haemogenic endothelium in the primitive yolk sac. That yellowish bag-looking thing that I always dissected and disregarded when doing my own experiments.
But Riley and his group didn’t stop there. Given the importance of the lymphatics in fluid homeostasis and inflammation, they were reasoned to have a role in cardiovascular disease, one of the biggest killers in the modern world. The team took lymphatic reporter mice and induced cardiac injury, by tying off a key artery supplying the myocardium. Lymphatic vessels expanded, and this growth occurs via the same programmes that drive lymphatic expansion in development. Using magnetic resonance imaging (which, as a medical student, I had no idea was possible in mice), they showed that treatment with a lymphangiogenic growth factor improved heart function after cardiac injury.
This paper isn’t my favourite solely because it challenged an age-old hypothesis, and did so robustly by using multiple parallel experimental strategies. It’s also the link it makes to a common disease process, and the manipulation the same programmes that drive lymphatic development to halt this process. This paper inspired me so much that I contacted Professor Riley to ask whether he had any free positions in this lab. Sadly, he didn’t. But, funnily enough, I am now tackling a PhD in lymphatic biology at my own university, integrated into my medical degree. And guess who I’m collaborating with!
References
- Klotz et al. (2015). Cardiac lymphatics are heterogeneous in origin and respond to injury. Nature, 522: 62-67.
Daniyal’s acceptance speech:
“I’m grateful to be receiving this prize from the BSDB, and I’m actually baffled I won, considering I’ve never had any formal teaching in developmental biology. I’m a medical student with no clear indication as to what clinical specialty I want to pursue, but with a strong interest in developmental biology. Unfortunately, I think I’m in the minority. I have lost count of the number of times that non-basic science-trained clinicians ask me about my interests, and when I respond, their faces screw up as if they have taken a large bite from a lemon.
Through my clinical career so far, I’ve always found myself coming back to developmental biology, with the support of the BSDB. First, as part of an integrated BSc degree during medical school, after which the BSDB supported my attendance at a conference in Chicago. Later in 2016, as part of a BSDB-funded summer studentship. And now, being supported by my medical school to do a PhD in lymphatic development, alongside my medical studies.
I think there is so much the clinical world has to learn from developmental biology, about birth defects, cancer, regenerative medicine and beyond. The paper I wrote about in my essay is just one incredible example of developmental biology’s potential. This paper, published in Nature by Paul Riley’s group in Oxford, showed that cardiac lymphatics develop in a completely unexpected way and, with two other papers published the same year, overturned a 100-year old dogma in lymphatic development. Riley and colleagues went on to show that tinkering with the same pathways that drive lymph vessel growth in development, can be used to manipulate lymph vessel growth to benefit cardiovascular disease. This is why it is undoubtedly my favourite scientific paper, since its publication in 2015.
I really hope, one day, I can forge a career that brings the clinical world and developmental biology closer together. So thanks to the BSDB and the massive support it’s provided me over the years, as you have given me hope that such a career can, and will, happen. And, of course, thank you again for this amazing prize.”
Painting the embryo by numbers: how nature provided the tools for an inspirational experiment (runner up)
Laura Hankins
Visit a local pond and lie flat on your stomach, allowing the soft mud to seep into your clothes. Be sure to bring a jam jar; it will sparkle in the lazy spring sunlight as you shift it closer to the water’s edge. Wait patiently, observing any disturbances to the dappled surface. There! The flat tail of a newt in the breeding season.
Pleurodelinae is an unassuming collection of newt species within the Salamander family. As a child, sitting by the pond in our front garden, I was often charmed by the sedate movements of common newts contrasting with the constant hum of traffic whipping past. I think I probably wanted to hunt for new species in undiscovered rainforests, as this seemed a reasonable career move at the time. Little did I know that the humble newt would reappear in a university lecture, starring in an experiment that inspired me to pursue Cell and Developmental Biology.
It is 1924. In Hans Spemann’s laboratory, our friends the newts have been the subject of a series of experiments performed by Hilde Mangold as part of her doctoral studies. Spemann was no stranger to amphibians; his work on eye development had made good use of frogs. Now he had turned his attention to how broader embryonic regions are defined.
During gastrulation, the embryo folds in on itself to produce three distinct layers that will ultimately have different fates. This produces the blastopore, an opening that acts like an insatiable mouth as the embryo consumes itself. Spemann, amongst others, had observed that transplanting tissue from the blastopore lip into another embryo resulted in the formation of a second neural tube and its surrounding structures. Many assumed that these features arose exclusively from the donor cells, but Spemann and others hypothesised that these cells could be acting as an ‘organiser’, signaling to influence their neighbours’ fates. But how to test this suggestion?
The breakthrough came with an idea that was beautiful in its simplicity. Mangold repeated the transplantation experiments but moved the tissue between different newt species. These newts had distinct pigmentations, so it would be possible to discern host from donor tissue after leaving the embryo to develop following surgery. In 1924, embryos left for sufficient time developed a chimeric conjoined twin with its own neural tube, notochord and somites. After sectioning, Mangold observed that these structures contained both pigmented and unpigmented cells. Remarkably, it seemed Spemann was right: the transplanted tissue had somehow altered the fate of the surrounding host cells, coopting them into forming an artificial twin.
This experiment is inspiring partly due to the minimalism of its approach; it demonstrates that the most influential experiments are designed without unnecessary embellishment. Yet its surgical element made it incredibly technically complicated. Thanks to their logical design, and the natural features of newts, Spemann and Mangold changed our perception of cell fate determination. Years later, researchers are still being inspired to use knowledge of the natural world to address questions at the cellular level.
Is the future of developmental biology written in science fiction? (runner up)
Victoria Rook
As a developmental biologist and devoted reader of dystopian science fiction, I frequently wonder how often these two things overlap, when will fiction become fact and fact mirror fiction? A couple of years ago I came across a fantastic book, Margaret Atwood’s Oryx and Crake 1. This book is set in a desolate, dystopian future that arose as a consequence of biotechnology corporations taking genetic engineering to extremes. The accountable corporations created many chimeric animals, one magnificently named example are the ‘pigoons’;
“The goal of the pigoon project was to grow an assortment of fool-proof human
tissue organs in a transgenic knockout pig host….1”
In January 2017, Juan Carlos Izpisua Belmonte at the SALK institute, California, published a paper in Cell titled; Interspecies chimerism with human pluripotent stem cells2. In this article, Belmonte introduced human stem cells into pre-implantation pig embryos. Chimeric human-pig embryos were then implanted into female pig recipients and developed for four weeks before analysis. The ultimate objective of this research is to grow replacement organs in pigs for human transplant. Unfortunately, Belmonte did not credit Atwood for her conceptual influence and, more disappointingly, there is no mention of ‘pigoons’ throughout the paper.
Atwood also describes a superior human species, the ‘crakers’, which were developed as prototypes of what could be available to those willing to pay top dollar for ‘genetically perfect’ children. A decade later, Feng Zhang’s group in Harvard were the first to report CRISPR-Cas9 mediated genome editing3. Shortly after, in 2015, Junjiu Huang’s group in China used this CRISPR-Cas9 technology to carry out targeted genome editing in human embryos4 with the intent to treat β-thalassemia. More recently, Kathy Niakan, a group leader at the Francis Crick Institute in London, has obtained a HFEA license to use CRISPR-Cas9 technology to manipulate the human embryo genome in order to study early development. Understandably none of these embryos have been, or will be implanted into human recipients, but how far away are we from being able to create Atwood’s ‘crakers’?
Oryx and Crake is one prophetic example where fiction has seemingly ‘foretold the future’, posing questions about how much can we learn about the future of developmental biology from science fiction? Aristotle showed us model organisms could be used to study development, however, given innovations in CRISPR-Cas9 technology and ongoing discoveries of evolutionary developmental differences between species, is it likely that model organisms will become a thing of the past and CRISPR-engineered human embryos pave the way of the future? Whilst this may sound like a pessimistic prognosis for the future of developmental biologists working with model organisms, I am actually very excited for what the future holds. Soon we will have the resources to cure numerous genetic diseases and, in theory, the ability to improve the lives and health of generations to come. The unease comes with how far are we liable to take this, is a dystopian future where ‘pigoons’ and ‘crakers’ run wild within our reach, or will they remain a thing of fiction?
References
- Atwood, M. Oryx and Crake. (Bloomsbury, 2003).
- Wu, J. et al. Interspecies Chimerism with Mammalian Pluripotent Stem Cells. Cell 168, 473–486.e15 (2017).
- Cong, L. et al. Multiplex genome engineering using CRISPR/Cas systems. Science (80-. ). 339, 819–824 (2013).
- Liang, P. et al. CRISPR/Cas9-mediated gene editing in human tripronuclear zygotes. Protein Cell 6, 363–372 (2015).
Does developmental biology have a future?
Anna Klucnika
Last year I marched the streets of London with thousands of other science enthusiasts, as many more thousands did so in other cities across the globe. The reason? Politics has gotten in the way of science and the public is, apparently, tired of experts.
With funding plummeting in the UK and already slashed in the US, the future of research is unclear. There is increasing pressure from the public, funding bodies and governments for biological research to focus on questions that generate click-bait headlines claiming a disease cause or cure. Research with direct applications for human health and disease is unequivocally important. However, recent funding trends reveal that basic biological research is being institutionally neglected. This will leave huge collections of questions unanswered. Many of these questions would have lead onto findings with broad implications that could have revolutionised human health.
Developmental biology is one such science that is often perceived to have little implication on the lives of the majority of the population. Developmental biology is the study of how cells make tissues, organs and organisms. This humble field has led to many discoveries with worldwide impact, for instance cloning, understanding birth defects and optimising IVF. Nevertheless, developmental biology is persistently undervalued. Research using animals that are more commonly known as pests was always going to be a hard sell.
In walks Developmental Biology’s sexier cousin: stem cell and organoid biology. Really, these two scientific fields are more likely sisters. Both ask the same question (how do cells make organs), but stem cell and organoid biology inherently has more of a focus on research with direct therapeutic applications. Organoids can be used to aid our understanding of organ growth and tumorigenesis, to screen for drugs and may potentially enable us to grow organs for transplantation and so is attractive to funders and, crucially, the public.
To grab the public’s attention, Developmental Biologists need to learn from the organoid field and showcase the research that has fast-track therapeutic potential. Old school developmental biologists whose careers blossomed when curiosity-driven science was enough will be severely offended by this statement. But scientific culture has dramatically changed. We are in an era of information overloaded in which headlines are updated every minute, not day. Sexy science thrives in this environment, whilst the less-glamorous fields are quickly forgotten.
To stay in the game, Developmental Biology needs a makeover. We need a new vocabulary that everyone can understand. We need to be proud of the research that will have an impact on the public’s life. Most importantly, we need to be united in our goal. Developmental biology is an extremely broad term. We must not be eager to divide ourselves based on our research, our question or our model. Ultimately we are all trying to work out the mystery of how a single cell can make something as amazing as a plant or animal. Once we remember that, we can share the sex appeal and developmental biology can thrive. Maybe we can convince people that experts aren’t so bad after all.
The future of Developmental Biology – addressing biological complexity
Kane Toh Qin
Conrad Waddington proposed the metaphorical epigenetic landscape in the 1940s as a model for the unfolding of discrete cell fates. In the modern post-genomic era, the metaphor has experienced a resurgence in popularity. Tailored with the theory of dynamical systems, developmental biologists have used the conceptual apparatus to make quantitative predictions of differentiation dynamics. The appeal of the epigenetic landscape illustrates the importance of conceptual frameworks in developmental biology to highlight general principles of development; a notion that is especially pertinent in light of the overwhelming complexity of the ‘omics’ datasets today.
As sequencing technologies, time-lapse imaging techniques and genetic engineering methods continue to improve, we will have the technical tools to probe the epigenetic landscape. I believe that Developmental Biology in the future will provide increasingly coherent and precise explanatory accounts of phenomenological discontinuities that arise in different spatial and temporal scales of organismal development. For instance, how do fluctuations in the number of mRNA and proteins in a single cell allow for the coordination of cellular decisions over cell populations? How is the genotype-to-phenotype map implemented in an organism, and how do these properties influence its long-term evolutionary dynamics and vice versa?
To pave the way for these explanations, developmental biologists should further appreciate that knowledge of the component parts of biological systems alone will not beget knowledge of principles of development. As the complex systems biologist Kunihiko Kaneko argues, using an analogy from physics, without the existence of (macroscopic) thermodynamics, statistical mechanics, which connects microscopic behaviour to macroscopic thermodynamic quantities, would not have existed as a branch in physics. This will encourage a shift in perspective away from a reductionistic snapshot of biological components to a processual, dynamic framework of developmental mechanisms as coupled, interacting processes in complex systems. As the physicist Robert Laughlin puts it affectionately, life is the ‘granddaddy of emergent phenomena’ and emergent phenomena, as we know it, arise from a collection of interactions.
The complexity of living systems is such that further progress in Developmental Biology will inevitably require advances that derive from interdisciplinary dialogues between natural scientists. As such, developmental biologists will become more familiar with the tools and concepts involved in constructing quantitative explanations to complement their study of developmental mechanisms. For example, information theoretic measures like entropy are used today by some biologists to understand the process of stem cell differentiation at a single cell level.
With the accumulation of so much biological data, one can sympathize with the notion that the complexity of biological development eludes further human understanding—a position that spurred the embryologist, Hans Driesch to embrace vitalism. But we should be optimistic about the future: organismal development can be understood mechanistically, by first extracting its most important features and then analysing the patterns that emerge with the interdisciplinary tools and conceptual frameworks at our disposal. The principal task of developmental biologists then is to continue directing attention to the Biology and working out the crucial biological features for investigation.
The experiment/paper in Developmental Biology that most inspired you
Emilio Mendez
My history is funny I think. When I was a kid, I remember asking my father about why elephants were so big? Why mice so small? Why our Pomeranian dog was small? Meanwhile, our neighbour had a big German Shepherd. My father was intrigued by my questions and decided to bring a complete juvenile encyclopaedia and tried to find the answers I was looking for inside it.
Those questions usually disappear quickly with traditional education, puberty, football and love. My father past away when I was sixteen years old, however, he left me many teachings about life, he always told me two things, first, try to understand what you cannot explain, usually its worth. Second, what a man can build, another one also can.
When I had to choose my path after high school, I decided to study Biochemistry, because I like it a lot biology and chemistry. However I was not familiarised with the work of a scientist, for me it was more like “I like that, and I do not like mathematics”, however, I have never been so wrong. Soon I got in touch with that other science, and my mind was amazed at the fact that they are interconnected in such a beautiful way that it is childish to think they walk separated ways in build our world.
When I was finishing my career, I decided to take a course called “Genetic Control of Development”, totally unaware of what it was about, but what I found was love at first sight. Suddenly all my questions, my early questions came to my mind, especially when I saw the paper of Ross G. Harrison 1924, he transplanted limb buds of two different species of salamanders, with the idea of study which factors or signals affect the proportions of tissues. As the title of the paper, unexpectedly, both limb buds developed to its average size (donor size). That shocking difference was all I was looking for; suddenly I realise my question about the size of an individual it has no answer yet, however many factors have been described since Harrison (and Twitty 1931).
Those papers are essential for me because they summarise the beauty of Developmental Biology, simple question with simple experiments and a fantastic result, which open new roads to explore how the life has developed in our world. Later on, during my PhD studies, I meet the magnificent book “On Growth and Form”, it is the perfect crystal of all the sciences working together to build a life. I believe that if every boy and girl could see this book, many of them will never lose their curiosity about our world.
These are some of the elements that guide me to developmental biology, they drive me back to my childhood, like an old forgotten melody that came up out of nowhere. Just to realise how brave my father was.
I think he was telling me to study what a man cannot build yet, the life.
The future of Developmental Biology
Amanda BergAs the human species expands its curiosity and desire to explore the unknown, the concept of inhabiting other planets does not seem too distant. As humans, we are naturally curious and have always had the desire to explore our surroundings. Colonisation of Mars, or even more distant planets, will rely heavily on the successful reproduction and growth of the human species. Currently, scientists are investigating the physiological role that gravity and space travel can play on our bodies, but for successful fertilisation and growth of foetuses in space, we will also need to start thinking about the effect of space flight and gravity on a developing embryo.
Xenopus laevis embryos have previously been sent to space and as a result they grew into abnormally developed larvae. They had longer tails, combined with smaller heads and bodies than the Earth-grounded comparisons. Their notochords were deformed, with resulting abnormal curvature of the spine, causing them to swim in backwards somersaults (Snetkova et al., 1995). It is possible that these defects are due to abnormal dorsal-ventral axis specification which is determined through cortical rotation, and is thought to rely on gravity.
Another experiment was also proposed to attempt to grow chicken embryos in space, but due to the complicated experimental design and short time-scale given to the project, it never went ahead (NASA, 1977).
More recently, 2-cell mouse embryos were sent into space, which went on to develop into healthy blastocysts (Chinese Academy of Sciences, 2016). This exciting result means human embryos may also be able to develop in space. It would be exciting to investigate whether these blastocysts could be implanted into female mice, and whether they can form healthy offspring.
We will also need to consider whether normal reproduction can occur in space. Fertilisation had occurred on earth for all of these experiments, and the animals were also not grown to full term. We will also need to consider the different gravitational fields found on other planets; how would an embryo look if it was conceived and fully developed on the International Space Station, where gravity is almost minimal? What about on Mars, where the gravity is weaker than on Earth?
It is inevitable that humans will attempt to colonise other planets, and to do so we must investigate the possibility of reproduction and development of embryos in space. We can only hope that healthy foetuses can develop, and that the growth of the human species is not inhibited by its own developmental biology.
References:
Chinese Academy of Sciences (2016) “Chinese scientists develop mammal embryos in space for first time”, accessed 01.03.2018 [LINK]
NASA (1977) “SP-401 Skylab, Classroom in Space. Chapter 5: Embryo Development in Space”, accessed 01.02.2018 [LINK]
Snetkova, E. et al. (1995) ‘Effects of space flight on Xenopus laevis larval development’, Journal of Experimental Zoology Part A, 273(1), 21-32
What has developmental biology contributed to society?
Sandra G Gonzalez Malagon
“How are we formed?” My 6-year old nephew asked me with innocent wisdom. It’s been 17 years since and I still do not have a simple answer for him. The observation of natural life and the attempts to explain the world around us has fascinated human beings throughout our existence. Even Ancient Greeks considered fundamental questions about embryogenesis and inheritance. Developmental Biology (DB) covers all of these related questions: How do different organisms develop? What controls the patterning of different species? What are the subtle differences that lead to individual characteristics? What happens if the synchrony is disturbed? How does the environment influence an organism’s development?
Our Society today has a better understanding on how a person’s life develops from the womb. New parents can not only envision the stages of development of their unborn baby, but they also are aware of the importance of reproductive age, a healthy lifestyle and the risks of alcohol, cigarettes, medications and environment on their child’s development. They can be offered in utero diagnosis of chromosomal abnormalities or assessment of embryos prior to implantation can be a choice for parents who present high risks of passing a disease to their child. This information has made a tremendous impact not just for families, but also potentially eradicating disease and addressing global health problems.
DB studies identified the factors required for a cell to remain pluripotent -undifferentiated state- and the factors to induce these cells into specific cell types, (muscle, neurons, etc.) These findings provided the basis for the complicated protocols used to culture them in vitro. The surprising ability of these pluripotent cells to self-organise ex vivo and differentiate into a functional “organoid” (a small replication of a functional organ) has given the field of translational and basic research an outstanding advantage. These organoids can be cultured from human cells and are a great tool to model human diseases and to find personalised treatments. Stem Cell Research and Regenerative Medicine are the two emerging fields in Biology that have reshaped the way we think of development and disease. Although not yet mature, potential treatments for diabetes, neuronal regeneration, or gut repair -just to mention a few- are in the list of possible successful treatments, that up to date, have not been found.
From understanding how we develop to finding disease treatments, how other organisms develop to how the environment influences these processes and evolution, DB will continue to be the “stem cell of biology disciplines”, as Professor Scott Gilbert described in his recent essay on this topic. DB has the potential to give rise to many disciplines in biology, as it has been doing for a long time. As for my nephew, I hope that the innate developmental biologist that lives in every kid continues to be curious and astonished about the natural world that surrounds us. Only this will help preserve our planet and the species that live in it.
The Duty of Developmental Biologists
Massimo Ganassi
“So, which illness are you trying to make the cure for?” -he asked me mumbling- “Well, we are now studying how muscle is formed during embryonic development and this may be helpful to understand what goes awry in muscle diseases and therefore suggest a cure”. Even more confused he looked at me and said: “ mmm… but I guess it would be better, and quicker, to study how to cure the disease directly rather than how something is working when is working right, isn’t it?”. I admit, this always sounds a very honest, genuine and reasonable suggestion from a “non-science” person. Indeed, at this point I usually avoid adding confusion saying that I do research on embryonic fish muscle development.Similar conversations happen frequently to me, whenever I try to explain what my job is and how scientists spend their hours working.
For everyone, from kids to older people, it is easy to appreciate the efforts of a baker, a plumber or a bus driver simply because they produce usage for everyone. In contrast, the importance of scientific research does not reach the many and Developmental Biology is still thought to be a mere scientific and academic discipline whose results are far away from everyday life needs. Sadly, this just reflects how far the “normal” and “scientific” worlds are still apart, and it is partly our fault.
From the pioneer work of D’Arcy Thompson1, through the golden decades of developmental biology, to the most recent “omics” era an incredible amount of knowledge has been reached and fruitfully contributed to our wellness. As insiders we all know that developmental biology deeply enhanced the understanding of a multiplicity of biological processes from fertilisation of egg cells to the healing of wounds, nevertheless contributing to the treatment of human conditions such as infertility, cancer or genetic diseases. Moreover, several excellent works have highlighted the central role of Developmental Biology in an attempt to attract and engage the lay public into scientific research discoveries2,3,4,5.
As a matter of fact, nowadays, communicating science is even more important than making scientific discoveries. This is also reflected by the importance given to public outreach events and their primary role even in grant proposal applications. We must convince people that basic research is worth their consideration and money donation. Recently, to help scientists in public outreach, many platforms have become available to spread science, from the more specialist web-forum The Node to the more common social media6.
We do have the responsibility and duty to translate the importance of our research by strengthening our communicative potential. This means explaining to people why animal models such as mouse, zebrafish, frog and fruitfly are so important in our research and how developmental biology has contributed to everyday life, from the most recent medicine advance to the latest agriculture technique. This has to be the main goal of every scientist. We do make daily efforts to achieve our research aims and we must make our goals and results understandable by everyone.
References
[1] Thompson D’AW. On Growth and Form. (1917) Cambridge University Press.
[2] Prokop A. What is developmental biology and why is it important. (2018) https://www.openaccessgovernment.org/developmental-biology-important/41386/
[3] Edge L. What Is the Future of Developmental Biology? (2017) Cell 170:6 -7, doi: 10.1016/j.cell.2017.06.019
[4] St Johnston D. The Renaissance of Developmental Biology. (2015) PLoS Biol 13(5): e1002149. doi:10.1371/journal.pbio.1002149
[5] Pourquié O. Development: looking to the future. (2012) Development 139: 1893-1894; doi: 10.1242/dev.082685
[6] Vicente C., Maartens A., Brown K. The Node and beyond-using social media in cell and developmental biology. (2017) Semin Cell Dev Biol. 70:90-97. doi: 10.1016/j.semcdb.2017.05.009.