BSDB Gurdon Summer Studentship Report – Stephanie Leadbitter
Posted by Stephanie Leadbitter, on 17 November 2021
Mechanical regulation of cell division in developing tissues: Speed Vs Strength
During embryogenesis, dynamic mechanical forces act on developing tissues, inducing cellular mechano-responses. These changes in cellular behaviours such as cell division, adhesion, and motility are a vital aspect of tissue morphogenesis and homeostasis.
This summer, I was given the opportunity to work under Dr. Woolner at the University of Manchester’s Division of Cell Matrix Biology & Regenerative Medicine. Using a multidisciplinary approach, Dr. Woolner’s lab examines the cellular response of developing tissues to an applied mechanical force and seeks to identify the underlying molecular basis. This placement was an incredible and unique opportunity for me, as I was able to receive training and experience in a variety of new techniques used in biomechanics, mathematics, biomodelling and developmental biology.
Previous work by the Woolner lab demonstrated that the rate of cell division increases in epithelial cells following the application of a low-magnitude, uniaxial tensile force1. Work in other systems has shown that similar mechanically-induced increases in proliferation occur due to upregulation of the ERK1/2 pathway downstream of the stretch-activated calcium channel Piezo1, culminating in an upregulation of cyclin B2. Additionally, it is known that the orientation of cell division aligns with the axis of stretch1.
However, all current studies investigating the cellular response to tensile force involve rapid, instantaneous tissue stretching. Under physiological conditions, changes to mechanical tension in the developing embryo occur over a period of minutes to hours rather than seconds. In tumourigenesis, mechanical changes may take place over years. It is not currently known how cell division rate differs between fast and slow stretch regimes. Preliminary work suggests that slow-stretch regimes may not elicit the same division responses that are seen with instantaneous stretching.
My project aimed to help shed light on whether the speed or strength of an applied mechanical force is the major factor in altering cell division rate.
Using a tissue stretching apparatus, we applied an instantaneous, uniaxial stretch with reduced strength to tissues. For these experiments, Xenopus laevis embryonic tissue was used. Xenopus laevis embryos are a robust model organism for use in biomechanical research as they are large, develop externally and are easily visualised. I was very grateful for the opportunity to shadow members of the lab working with the Xenopus colony throughout the project. They are a unique model animal (I also have a few as pets!) and it was great to see how they are cared for and used responsibly in a research setting.
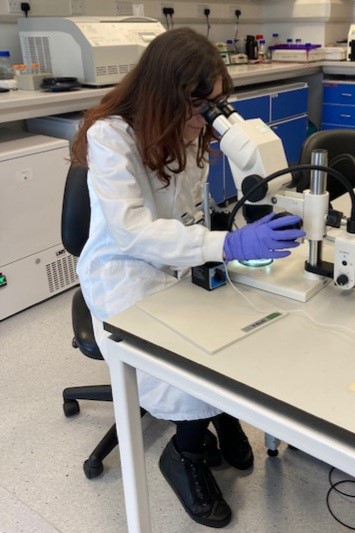
Fig 1. Selecting embryos at 2-cell stage for mRNA microinjection
In order to visualise the cell edge and nucleus, Xenopus embryos were injected at 2-cell stage with GFP-tubulin and Cherry-histone RNA. Straight away I was given the chance to jump in and get involved with the experiments, as I helped Gina (the Woolner Lab’s Research Assistant) with DNA miniprep and mRNA preparation. We proceeded with microinjection, which involved inserting a microscopic needle tip into each cell under an optical microscope. This was a very tricky procedure at first but by practicing alongside Gina, I was eventually able to go from struggling to inject 10 embryos in an hour to injecting over 50 in half the time!
Following overnight incubation, embryos were staged at early gastrula and the animal caps were dissected. Isolated animal cap explants are a versatile tissue able to survive and develop ex-vivo, making them ideal for live imaging. Dissecting the animal cap was done through an optical microscope using two sets of forceps. This was the most technically challenging aspect of my lab work, as it required a steady hand and patience but couldn’t be done too slowly or the embryos would become too developed. It was very rewarding to eventually get a perfect set of animal cap explants.
Following incubation on a fibronectin-coated silicone membrane, the animal caps were stretched and imaged. Shown in Figure 2 is a single frame from one of our live movies captured using confocal fluorescence microscopy. This was great experience, as imaging science was always of great interest to me but I had never previously had the chance to put my theoretical knowledge into practice. I also used image analysis software to calculate the mitotic index, as well as try cell population tracing. The Woolner lab uses tracing alongside vertex modelling3,4 to measure cell shape and infer mechanical stress across the tissue. The data collected during my project will be used to determine whether an increased cell division rate acts to relieve tensile stress across the tissue.
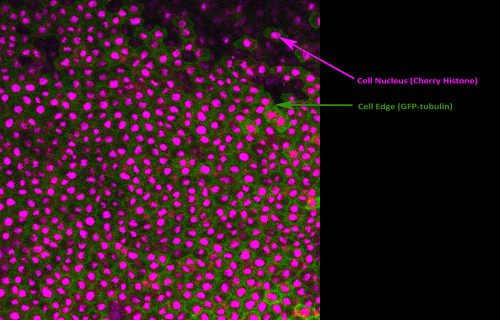
Fig 2. Fluorescent image of a Xenopus embryo animal cap explant experiencing a uniaxial stretch. Visualisation of the cell nucleus (magenta) and cell edge (green) allows image analysis techniques and cell population tracing to be performed. This was performed to calculate the mitotic index and biophysical properties of the tissue.
Alongside my core project work, I also successfully titrated the CDK-1 inhibitor RO-3306 to find the optimal concentration for cell division inhibition in Xenopus embryos. It is currently known that mechanical tension may increase cell division in fast-stretch regimes by promoting G1 to S phase transition5, which the Woolner lab will be investigating in slow-stretch regimes using a Fucci probe coupled with RO-3306 inhbition. Towards the end of my studentship, I was really grateful to have the opportunity to attend the 18th International Xenopus Conference. This was a great chance to discover the wide array of biomedical research using Xenopus currently being conducted worldwide and make valuable connections.
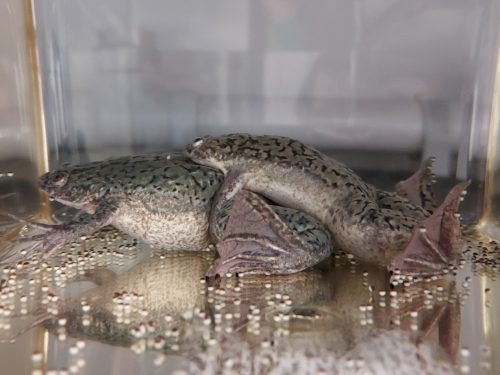
Fig 3. Xenopus laevis produce large, externally developing embryos which are easy to collect, visualise and manipulate. These properties make them particularly suitable for tissue stretch experiments.
I would like to thank everyone for all their support, guidance, patience and coffee & cake sessions throughout the internship. I am very grateful that I was able to receive the Gurdon/BSDB Summer Studentship and would recommend any student interested in developmental biology research to apply. Gaining first-hand lab experience in this field has given me invaluable skills and insight and has opened many doors for my future career.
References
- Nestor-Bergmann A., Stooke-Vaughan G.A., Goddard G.K., Starborg T., Jensen O.E. and Woolner S. (2019) Decoupling the roles of cell shape and mechanical stress in orienting and cueing epithelial mitosis. Cell Reports 26: 2088-2100
- Gudipaty S.A., Lindblom J., Loftus P.D., Redd M.J., Edes K., Davey C.F., Krishnegowda V., Rosenblatt J. (2017) Mechanical stretch triggers rapid epithelial cell division through Piezo1. Nature 543, 118-121.
- Nestor-Bergmann A., Goddard G., Woolner S. and Jensen O.E. (2017) Relating cell shape and mechanical stress in a spatially disordered epithelium using a vertex-based model. Mathematical Medicine and Biology 35 (Supplement 1): 1-27
- Jensen O.E., Johns E. and Woolner S. (2020) Force networks, torque balance and Airy stress in the planar vertex model of a confluent epithelium. Proceedings of the Royal Society A 476: 2237
- Benham-Pyle B.W., Pruitt B.I and Nelson W.J. (2015) Mechanical strain induces E-cadherin-dependent Yap1 and β-catenin activation to drive cell cycle entry. Science 348(6238): 1024–1027.