The people behind the papers – Julia Brandt, Mary Rossillo and Niels Ringstad
Posted by the Node Interviews, on 9 May 2019
This interview, the 61st in our series, was recently published in Development
A fundamental aim in developmental biology is to understand how the various cell types of the body are specified by differential gene regulation. Caenorhabditiselegans nervous system development provides a powerful system for studying this, as exemplified by a new Development paper reporting on how the BAG neurons that help the worm sense oxygen and carbon dioxide are specified. We caught up with first authors Julia Brandt and Mary Rossillo and their supervisor Niels Ringstad (Associate Professor at the Skirball Institute of Biomolecular Medicine and Department of Cell Biology at New York University) to find out more about the story.
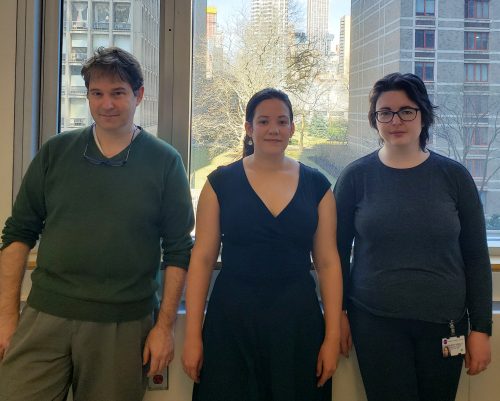
Niels, can you give us your scientific biography and the questions your lab is trying to answer?
NR I’ve had a longstanding interest in molecular neurobiology. This started during my graduate studies with Pietro De Camilli at Yale University, where I studied molecules required for the synaptic vesicle cycle. During my postdoctoral training with Bob Horvitz at the Massachusetts Institute of Technology I was introduced to behavioural genetics of C. elegans as a method to identify molecular mechanisms that are required for the function of specific neuron-types or for specific neurotransmitter signals. My own group continues to study the genetic basis of C. elegans behaviours to advance understanding of neuromodulation and sensation.
Julia and Mary: how did you each come to join the Ringstad lab, and what drives your research?
JB I’ve always been interested in how specific cells acquire the characteristics that distinguish them from other cells. The nervous system is where this question really stands out. There are hundreds of kinds of neuron, and each kind has a unique morphology and distinctive physiology.
MR I was drawn to the lab by the possibility of using C. elegans to understand how neurons sense respiratory gases. The worm gas-sensing neurons are specified by a transcription factor called ETS-5, which has a vertebrate homologue that is required to specify neurons in the mammalian brainstem that sense respiratory gases in arterial blood. I thought that this similarity offered the opportunity to leverage techniques in developmental genetics to interrogate mechanisms that might be involved in human pathology, specifically fatal respiratory disorders like Sudden Infant Death Syndrome. Because I am training to be a physician-scientist, I wanted my graduate research to impact clinical medicine.
Why are the BAG neurons an interesting developmental model, and what was known about their specification before your work?
NR We have colleagues studying chemosensation or neurodevelopment who previously identified and characterized mutants that are defective in BAG development. Elissa Hallem at the University of California, Los Angeles, had identified the ETS-5 transcription factor through an independent study. Roger Pocock at Monash University in Australia collaborated with us in our study of ETS-5, and his group independently identified two other transcription factors that are important for BAG development, EGL-13 and EGL-46. From this work, we had the sense that the genetics of BAG neuron development would be complicated and rich. Indeed, our own genetic screens yielded mutants that did not come up in Elissa’s or Roger’s studies. Our screens also yielded a mutation that causes ectopic expression of a BAG fate – a phenotype that is the mirror-image of the more common absence-of-BAG fate phenotype. This mutation was the starting point for the work that Julia and Mary did.
JB The BAG neurons exhibit some remarkable physiological characteristics, and they have an interesting and easy-to-measure function. BAGs are able to sense the respiratory gas carbon dioxide as if it were an odour. The carbon dioxide that they sense is coming from environmental microbes, which the worms eat. We think that worms prefer to eat dead microbes, or microbes that are growing slowly, and BAGs are used to sense whether microbes are metabolically active. I think that the development of these neurons is interesting because understanding how the BAGs are put together can help us understand how they do what they do.
MR I agree with Julia. The BAG neurons are an interesting developmental model because they have remarkable physiology that is imparted by a developmental programme. And that programme requires a transcription factor, which is conserved between worms and humans. I like the idea that, by studying the developmental mechanisms that make a BAG neuron a BAG neuron, we can identify molecules required for its chemosensory function.
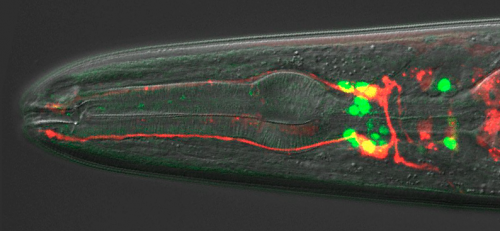
NR I agree with Julia and Mary. Understanding the developmental programme that generates a BAG neuron is going to teach us how these neurons execute a pretty cool function. And we now have the opportunity to study how that developmental programme is initiated and organized, which is new territory for us.
Can you give us the key results of the paper in a paragraph?
JB, MR & NR Chemosensory BAG neurons of C. elegansrequire the transcription factor ETS-5 to express a host of genes that are required for their specialized function. How expression of ETS-5 is regulated during development was unknown. Julia and Mary have found that ETS-5 expression is regulated by another transcription factor – VAB-3 – which is the nematode homologue of Pax6/Eyeless. Remarkably, the vab-3 locus encodes VAB-3 isoforms that have opposing functions with respect to regulating expression of a BAG fate. One isoform represses expression of ETS-5 in cells that are not supposed to become BAGs. Another isoform, however, is expressed in BAGs and is required for them to fully differentiate. Julia and Mary concluded that the decision to express one VAB-3 isoform or another is crucial for determining which cells become BAG neurons.
Have you got any ideas what controls which vab-3 isoform is expressed in a given cell?
JB, MR & NR That’s the key question! We had a short-lived feeling that we had learned something when we realized that ETS-5 expression was regulated by VAB-3. But now we feel like we have simply re-framed the question as one that concerns promoter-selection in the vab-3 locus. But we’re not completely in the dark, and we now have some ideas about what is going on at the vab-3 locus. Mary has been pushing hard to establish methods for purifying and sequencing chromatin associated with sparsely expressed transcription factors, and some of her studies are identifying factors that bind to vab-3 promoters. Stay tuned.
Why might the system of broad repression/selected activation of cell fates have evolved in animal development?
JB, MR & NR Our first thought is that biological systems aren’t designed or engineered, so we shouldn’t be surprised when we discover that they are rife with needless complexity and inefficiency. During evolution, a set of transcriptional repressors and activators might have been randomly permuted until they generated a pattern of ETS-5 expression that worked. We might be looking at the mess that was left behind in that workshop. But it is also possible that we are looking at a legacy of an ancestral and now-obsolete developmental programme. Cells with the characteristics of BAG cells might have been more widespread in the ur-nematode, and over time more efficient body plans that used those cells for other purposes became the norm. A final thought we have entertained is that there is some drive to carefully regulate expression of a BAG fate. A good control system will use both positive and negative regulators of its output, which allows rapid but controlled responses to inputs.
When doing the research, did you have any particular result or eureka moment that has stuck with you?
JB: Finding a mutant is always a eureka moment. I had found a large number of mutants that lacked expression of a BAG marker. It was pretty surprising to find a mutant with extra cells expressing that marker. And it was exciting to discover that the phenotype was caused by ectopic expression of ETS-5.
MR Julia had found that vab-3 mutated to cause ectopic expression of ETS-5, and she had tested a number of vab-3 alleles. We had the idea that one isoform was repressive and the other not, but we were confused by reports that vab-3 is highly expressed in BAG neurons. It was satisfying to design isoform-specific reporters and see that the long repressor isoform was not expressed by BAGs. This really crystallized our model of a promoter-selection event that patterns expression of a BAG fate.
Finding a mutant is always a eureka moment
And what about the flipside: any moments of frustration or despair?
JB & MR: Some of the mutants we used are difficult to work with because they are barely viable or the mutations cause lethality. ceh-32 was awful. It is an essential gene, but we also found that ceh-32 reporter transgenes could cause lethality. We had to figure out how much DNA to use to make the ceh-32::GFP trangenics that we needed for the project.
So, what is next for you two after this paper?
JB I’m wrapping up a post-doc and deciding on the next steps for my career.
MR I am halfway through my dissertation research. Now I’m focusing on using mRNAseq and ChIPseq to generate a genome-wide map of ETS-5 targets. I’m hoping this will lead to novel genes that function in carbon dioxide sensing.
Where will this work take the Ringstad lab?
NR We’re still trying to link the developmental programmes that specify a BAG cell to genes required for gas-sensing. Julia and Mary have put another transcription factor on the board – the isoform of VAB-3 that promotes a BAG fate. I think we want to know how that factor functions together with ETS-5 and what genes it regulates.
Finally, let’s move outside the lab – what do you like to do in your spare time in New York?
JB I enjoy life in Brooklyn, spending time with my family and reading.
MR I enjoy distance running, yoga and spending time with my cat, Ernie.
NR Family life, home repairs, and I’m trying to catch up on all the maths and physics I didn’t do at university because I was too preoccupied with biology.