A day in the life of a larval-microbe lab
Posted by tjcarrier, on 21 March 2017
Hello, my name is Tyler Carrier and I am a second year PhD student in the laboratory of Adam Reitzel at the University of North Carolina at Charlotte. Broadly speaking, this laboratory focuses on the ecology, evolution, and development of marine invertebrates and their life history stages. The basis of this work uses echinoderms and cnidarians, with most efforts pertaining to the intertidal starlet sea anemone Nematostella vectensis. I, however, have gone against this common thread and work with larval stages of echinoids (sea urchins); namely, the contribution of associated-microbiota in their development, evolution, and response to environmental variation.
Echinoids and their larvae
Sea urchins are part of the Phylum Echinodermata (Class: Echinoidea) and are close relatives to sand dollars (Echinoidea), sea stars (Asteroidea), brittle stars (Ophiuroidea), sea cucumbers (Holothuroidea), and feather stars (Crinoidea). Sea urchins are a group of bottom-dwelling (benthic) invertebrates found throughout the world’s ocean and are the primary grazers of sub-tidal kelp beds. In many cases, urchins spawn annually by releasing gametes into the water column where fertilization and larval development take place.
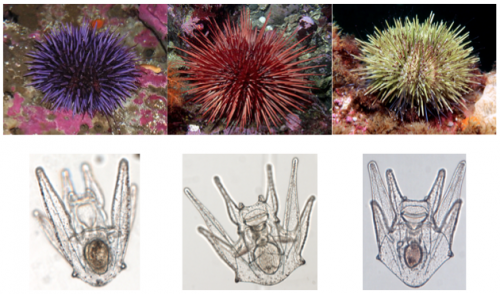
Urchin larvae, termed the echinopluteus, develop in the water column for weeks to months, during which they are at the mercy of ocean currents and, as a result, may be transported great distances from their parental origin. While developing the echinopluteus faces a multitude of environmental variants, including salinity, temperature, and food quantity. Over the course of evolutionary time, the echinopluteus has coped with these variants by altering their physiology and developmental trajectory.
My dissertation aims to shed light on if and how associated microflora provide larvae the genomic compliment to cope with abiotic and biotic environmental variables. Microbes associated with the animal host often differ considerably in the laboratory from that in nature. Therefore, to most accurately assess my research questions, I journey from the comforts of my bench to the field, where I collect and spawn adult urchins and rear their larvae.
A typical day in the field
Days in the field do not, in any way, reflect those at the bench. Time in Charlotte is consumed by processing samples: DNA extractions, PCRs (and re-doing PCRs because of contamination – there are non-larval bacteria everywhere!), gel electrophoresis, using QIIME and R to analyze 16S rRNA datasets, and writing grants and manuscripts. When compared to the field, those days are boring! And, as with many field-oriented biologists, the few weeks spent in the field dictate productivity for the year. Below I will outline a day from recent fieldwork at the Friday Harbor Laboratories (University of Washington), a field station located on an island wedged between the Olympic Peninsula and Vancouver Island.
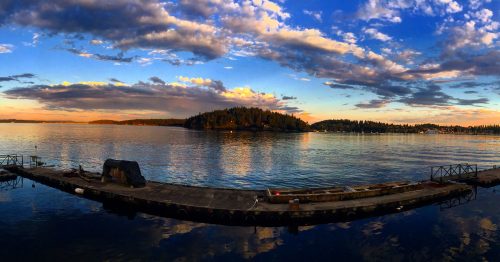
06:00: My alarm goes off, I stumble out of bed, and, as it was the day before, find that the sun is just rising. To start the day refreshed, I shower and head to breakfast.
07:00: Prior to caring to my larvae, I make a list of what must be done today and another for what I’d like to do if I find some spare time. The first list typically includes a tally of which cultures of larvae need to be sampled, imaged on the resident compound microscope, and need a water change and to be fed. Depending on the day or week, I tend to note that adult urchin cultures need feeding (incase I need more larvae) and to do so need to get in a row boat and collect drift kelp around the dock.
07:30: I begin by using a hemocytometer to approximate the density of my phytoplankton cultures (for feeding larvae).
08:00: One by one, I reverse filter larval cultures (three liter glass jars) down to ~100 mL. Each takes approximately ten minutes, and, depending on the day, I filter 12 or 24 cultures.
After each jar is reverse filtered, I transfer the remaining seawater to a finger bowl and, using a dissecting microscope, count ~100 larvae and transfer these to a 1.5 mL pre-labeled Eppendorf tube. This is one of the most important steps and focal points because here I am able to survey larval health, and am able to make anecdotal observations that may be interwoven with empirical results.
Following reverse filtration and sampling, larvae caged in Eppendorf tubes are spun into a pellet and seawater is replaced with a fixative (RNAlater) to preserve most DNA and RNA. These fixed samples are then moved into a -20 °C freezer.
12:30: With sampling complete, it is time to break for lunch and to catch up with resident and visiting scientists, their students, and those taking summer courses.
13:30: Having been fed, it is time to make sure the larvae are, as well. Based on phytoplankton counts this morning, I subsample phytoplankton cultures and allocate food quantities to their respective jars.
14:00: Now that husbandry is done, it is time to image larvae from each treatment. A small aliquot of larval cultures are transferred to finger bowls and are brought to the resident compound microscope.
16:00: To clear the mind from a taxing daytime session and to start de novo for the nighttime shift, I trade my laboratory attire for running gear and explore the island in a 45-minute to an hour run before dinner. Although this may take away from research time, it allows the rest of the day to be more focused and productive. Plus, the views aren’t half bad!
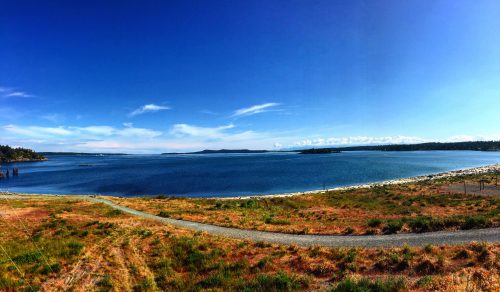
18:00: To be able to distinguish larval-specific microbes from environmental microbes, it is essential to sample the seawater, as well. While waiting, this semi-free time allows me to read a paper or two.
19:30: To monitor the effect of my experimental treatments on larval growth, the evening begins by analyzing the images taken before dinner. For this I use ImageJ and a cup of coffee. Hey, at least the larvae are adorable!
22:00: One activity that hasn’t been featured yet is e-mail and writing, and that’s because larvae take priority. To conclude the day, I catch (back) up on e-mail and try to write a couple paragraphs for upcoming grants and manuscripts in preparation. This takes me until about mid-night, by which time I should make my way towards bed… but tend to get distracted with a game or two of billiards or ping-pong.
00:30: Until tomorrow…